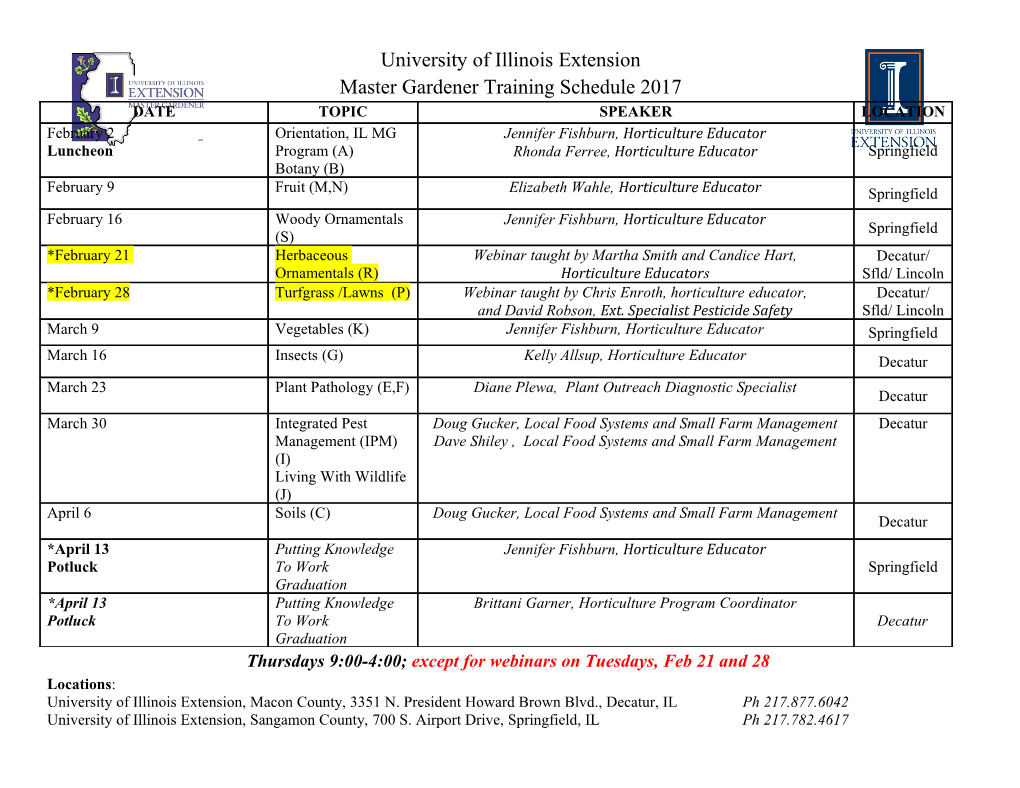
Structure and Mechanism of the Formylglycine-generating Enzyme By Mason J. Appel A dissertation submitted in partial satisfaction of the requirements for the degree of Doctor of Philosophy in Molecular and Cell Biology in the Graduate Division of the University of California, Berkeley Committee in charge: Professor Carolyn R. Bertozzi, Co-Chair Professor Judith P. Klinman, Co-Chair Professor Jack F. Kirsch Professor Matthew B. Francis Fall 2017 Structure and Mechanism of the Formylglycine-Generating Enzyme © 2017 By Mason J. Appel Abstract Structure and Mechanism of the Formylglycine-generating Enzyme By Mason J. Appel Doctor of Philosophy in Molecular and Cell Biology University of California, Berkeley Professor Carolyn R. Bertozzi, Co-Chair Professor Judith P. Klinman, Co-Chair The ability of enzymes to make rapid the conversion between chemical entities represents an enabling feature for life. The strategies by which enzymes achieve this feat are manifold in their details and physiological consequences. Though the central importance of biological catalysis has been known for well over a century, the true breadth of enzyme function is still beginning to be appreciated. A fundamental description of enzyme catalysis is essential to understanding biological processes, and is also necessary in order to realize the promise of engineered enzymes for transforming medicine and manufacturing. The formylglycine-generating enzyme (FGE) is responsible for activating sulfatase enzymes in aerobic organisms by catalyzing the post-translational modification of a cysteine residue to formylglycine. Formylglycine permits sulfatase activity by acting as a covalent cofactor. Thus, the diverse roles of sulfatases in both animal and microbial biology underscore the importance of FGE. However, early investigations made clear that this enzyme operates by a novel mechanism with a unique protein fold. In turn, elucidation of the details of this biochemical transformation have remained enigmatic for almost two decades. This dissertation describes the structural and biochemical interrogation of FGE in pursuit of understanding its catalytic mechanism. Chapter 1 places into context the physiological importance and biochemical curiosities of formylglycine and FGE. In Chapter 2, I present the first structural and spectroscopic characterization of copper-bound FGE, defining it as a novel enzyme with intriguing features related to function. Finally, Chapter 3 reveals detailed structural and kinetic proof for several critical steps of catalysis by FGE, allowing the formulation of a mechanistic framework for further investigation. 1 This dissertation is dedicated to my mother and father. i Structure and Mechanism of the Formylglycine-generating Enzyme Table of Contents List of Figures iv List of Tables v List of Schemes v Acknowledgements vi Chapter 1. Formylglycine, a posttranslationally generated residue with unique catalytic capabilities and biotechnology applications 1 Introduction 2 fGly is an essential posttranslation modification of Type I sulfatases 3 fGly participates directly in sulfate ester hydrolysis 5 FGE catalyzes fGly formation and is dysfunctional in MSD 8 Mammalian FGE activity is regulated by protein partners 9 FGE defines a novel protein fold 11 FGE is a unique cysteine-dependent oxidase/oxygenase 11 anSME catalyzes O2-independent fGly formation on Ser/Cys sulfatases in anaerobes 14 Some organisms have as yet unidentified fGly producing systems 16 fGly provides a genetically encoded, site-specific protein bioconjugation handle 16 Conclusion 19 References 19 Chapter 2. Structural and spectroscopic characterization of the formylglycine-generating enzyme defines a singular class of copper-dependent oxidase 29 Introduction 30 ii Results 31 X-ray crystal structure of the copper-bound S. coelicolor FGE holoenzyme 31 Unique spectroscopic features of a transient Cu(II)-FGE complex 34 Insight into the mechanism of Cu(II)-FGE autoreduction 36 Redox preference of the resting enzyme 37 X-ray absorption spectroscopy of Cu(I)-scFGE 38 Discussion 40 Materials and Methods 46 References 50 Chapter 3. Insights into the mechanism of FGE: elucidation of a Cu(I)-coordinated enzyme-substrate complex, and discovery of an intermediate during catalysis 55 Introduction 56 Results 57 X-ray absorption spectroscopy of the enzyme substrate complex 57 Identification of selenocysteine as an alternative substrate for FGE 60 Measurement of a substrate deuterium kinetic isotope effect in scFGE 64 Detection of an intermediate by stopped-flow UV/vis spectroscopy 66 Single-turnover kinetic analysis of FGE reactions 68 Discussion 70 Materials and Methods 75 References 81 Appendix 86 iii List of Figures Figure 1.1 Overview of Type I sulfatase structure and fGly-mediated catalysis 3 Figure 1.2 Regulation and trafficking of mammalian FGE by protein partners 10 Figure 1.3 FGE structure, overall reaction, and speculative mechanistic proposal 12 Figure 1.4 Mechanism of fGly biogenesis by the anaerobic sulfatase maturing enzyme 15 Figure 1.5 Principle and application of aldehyde tag engineering 17 Figure 2.1 Crystal structure of copper-bound S. coelicolor FGE 32 Figure 2.2 Characterization of a transient Cu(II)-scFGE complex by UV/vis spectroscopy 34 Figure 2.3 The transient Cu(II)-scFGE complex is autoreduced to Cu(I)-bound scFGE 36 Figure 2.4 Oxidative dimerization of apo-FGE following Cu(II) addition 37 Figure 2.5 Single-turnover activity assays of Cu(I)- and Cu(II)-loaded scFGE 38 Figure 2.6 X-ray absorption spectra of Cu(I)-scFGE 39 Figure 2.7 A redox-dependent copper binding pathway 42 Figure 2.8 Structural comparison of diverse protein copper centers 44 Figure 2.9 Proposed mechanisms of FGE catalysis 45 Figure 3.1 Structure of potential FGE copper-oxygen complexes capable of performing H-atom abstraction from substrate 56 Figure 3.2 Structure of FGE peptide substrates used in this study 57 Figure 3.3 X-ray absorption spectra of the cuprous enzyme-substrate complex 58 Figure 3.4 The FGE copper center undergoes a shift in coordination environment upon substrate binding 60 Figure 3.5 Selenocysteine inhibits FGE activity toward the native cysteine substrate 62 Figure 3.6 pH-rate profiles for the conversion of cysteine and selenocysteine substrates to fGly 63 iv Figure 3.7 Proposed model for catalytic discrimination between cysteine and selenocysteine substrates 64 Figure 3.8 Steady-state kinetic analysis of heavy and light FGE substrates 65 Figure 3.9 Detection of an intermediate by stopped-flow UV/vis absorption spectroscopy 67 Figure 3.10 Kinetic isotope effect observed for decay of the 420 nm intermediate 68 Figure 3.11 Single-turnover kinetic analysis of FGE substantiates the 420 nm intermediate 69 Figure 3.12 Revised mechanistic proposal for FGE 73 List of Tables Table 2.1 Crystallography data collection and refinement statistics 33 Table 2.2 Cu(I)-scFGE EXAFS Fitting Results 40 Table 3.1 EXAFS Fitting Results for Cu(I)-scFGE and Cu(I)-scFGE-scP14 59 Table 3.2 Activity of scFGE toward cysteine and selenocysteine substrates 61 Table 3.3 Kinetic parameters of scFGE with H-scP14 and D2-scP14 substrates 65 List of Schemes Scheme 3.1 Synthesis of Fmoc-Sec(bzl)-OH 61 Scheme 3.2 Hypothetical kinetic mechanism of FGE 70 v Acknowledgements Completing graduate school under the mentorship of my advisor, Professor Carolyn R. Bertozzi, has been without a doubt the foremost privilege of my life. I thank her immensely, but it is inadequate; I owe Carolyn an innumerable debt of gratitude for her support over so many years. She welcomed me into her lab, and provided me with opportunities that I could have never imagined. She has been unfailingly optimistic, understanding, and inspiring. Even within my comparatively brief tenure in the lab, watching her perpetually strive, and usually reach, the next level has been remarkable. Her unparalleled intellect is matched by her penchant for facing new challenges, and her absolute dissatisfaction with the comfort offered by the status quo. Being among those who moved with the Bertozzi lab to its new home at Stanford University was an unexpected but ultimately enriching experience. In preparation for the move, Carolyn tirelessly worked to ensure that our institutional transition be as seamless as possible, and was more than sympathetic to the concerns that the move presented to myself and others. Though I missed my life at UC Berkeley, being welcomed into the community at Stanford has left me feeling very fortunate to have had two exceptional campuses to call home during graduate school. Of course, Carolyn’s fearless attitude made this possible. It is truly bittersweet to finish a momentous journey guided by, and in witness to, Carolyn’s passion, vision, and ambition. I would also like to thank my Co-Chair, Professor Judith P. Klinman for her care and mentorship beginning from our very first meeting. Pursuant to my interest in enzymes, Judith graciously invited me to join her group meetings every Monday morning. These self-effacing discussions exposed me to the profound problems of protein science, which also happen to be quite central to life and its origin. Her brilliance, enthusiasm, and creativity have, in no small part, inspired in me a deep captivation with the fundamental questions in biochemistry, and convinced me of the satisfaction achieved through pursuing their answers. Her kindness and generosity have been touching, and throughout my life, I hope to carry with me the same curiosity for Nature that she possesses. The completion of this dissertation would not have been possible without contributions from my collaborators. I thank Katlyn Meier for being a bright and talented scientist whose commitment has helped me surmount the greatest obstacles I have confronted in research. Her specialized knowledge and aptitude for applying it to new scientific problems has been remarkable. I feel very lucky to have worked with her, and under the guidance of her mentor Professor Ed Solomon. I also thank Julien Lafrance-Vanasse for his amazing contributions, and regret that our circumstances allowed only a short period to work together.
Details
-
File Typepdf
-
Upload Time-
-
Content LanguagesEnglish
-
Upload UserAnonymous/Not logged-in
-
File Pages108 Page
-
File Size-