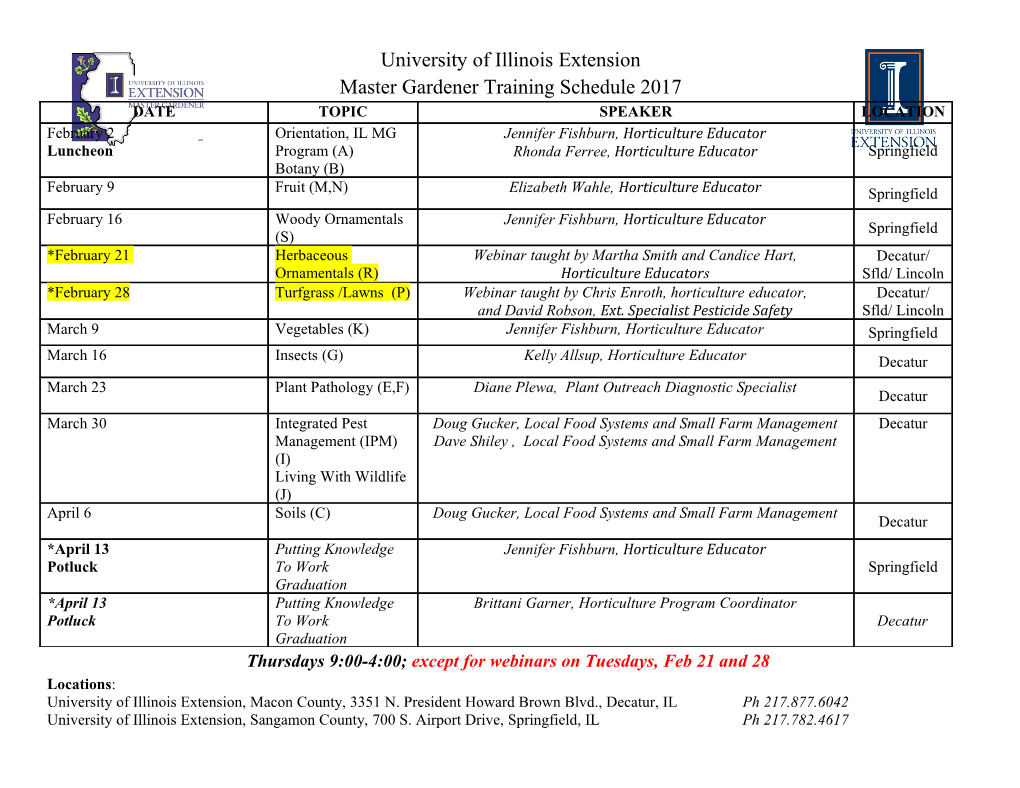
Biogeosciences, 18, 3505–3527, 2021 https://doi.org/10.5194/bg-18-3505-2021 © Author(s) 2021. This work is distributed under the Creative Commons Attribution 4.0 License. Geographic variability in freshwater methane hydrogen isotope ratios and its implications for global isotopic source signatures Peter M. J. Douglas1, Emerald Stratigopoulos1,a, Sanga Park2, and Dawson Phan1 1Earth and Planetary Sciences, McGill University, Montreal, H3A 0E8, Canada 2Department of Chemistry, McGill University, Montreal H3A 0B8, Canada anow at: Department of Earth Sciences, University of Toronto, Toronto, M5S 3B1, Canada Correspondence: Peter M. J. Douglas ([email protected]) Received: 5 November 2020 – Discussion started: 13 November 2020 Revised: 17 May 2021 – Accepted: 18 May 2021 – Published: 11 June 2021 Abstract. There is growing interest in developing spatially regions. We estimate a flux-weighted global freshwater δ2H– resolved methane (CH4) isotopic source signatures to aid in CH4 of −310 ± 15 ‰, which is higher than most previous 13 geographic source attribution of CH4 emissions. CH4 hy- estimates. Comparison with δ C measurements of both CH4 2 2 drogen isotope measurements (δ H–CH4) have the potential and CO2 implies that residual δ H–CH4 variation is the re- to be a powerful tool for geographic differentiation of CH4 sult of complex interactions between CH4 oxidation, vari- emissions from freshwater environments, as well as other mi- ation in the dominant pathway of methanogenesis, and po- 2 crobial sources. This is because microbial δ H–CH4 values tentially other biogeochemical variables. We observe a sig- 2 2 are partially dependent on the δ H of environmental water nificantly greater distribution of δ H–CH4 values, corrected 2 2 (δ H–H2O), which exhibits large and well-characterized spa- for δ H–H2O, in inland waters relative to wetlands, and sug- tial variability globally. We have refined the existing global gest this difference is caused by more prevalent CH4 oxida- 2 2 relationship between δ H–CH4 and δ H–H2O by compil- tion in inland waters. We used the expanded freshwater CH4 2 ing a more extensive global dataset of δ H–CH4 from fresh- isotopic dataset to calculate a bottom-up estimate of global 2 13 water environments, including wetlands, inland waters, and source δ H–CH4 and δ C-CH4 that includes spatially re- rice paddies, comprising a total of 129 different sites, and solved isotopic signatures for freshwater CH4 sources. Our 2 2 compared these with measurements and estimates of δ H– bottom-up global source δ H–CH4 estimate (−278 ± 15 ‰) 13 13 H2O, as well as δ C-CH4 and δ C–CO2 measurements. is higher than a previous estimate using a similar approach, as 2 2 We found that estimates of δ H–H2O explain approximately a result of the more enriched global freshwater δ H–CH4 sig- 2 42 % of the observed variation in δ H–CH4, with a flatter nature derived from our dataset. However, it is in agreement 2 slope than observed in previous studies. The inferred global with top-down estimates of global source δ H–CH4 based on 2 2 δ H–CH4 vs. δ H–H2O regression relationship is not sensi- atmospheric measurements and estimated atmospheric sink tive to using either modelled precipitation δ2H or measured fractionations. In contrast our bottom-up global source δ13C- 2 δ H–H2O as the predictor variable. The slope of the global CH4 estimate is lower than top-down estimates, partly as a 2 2 13 freshwater relationship between δ H–CH4 and δ H–H2O is result of a lack of δ C-CH4 data from C4-plant-dominated similar to observations from incubation experiments but is ecosystems. In general, we find there is a particular need for different from pure culture experiments. This result is con- more data to constrain isotopic signatures for low-latitude 2 sistent with previous suggestions that variation in the δ H microbial CH4 sources. 2 of acetate, controlled by environmental δ H–H2O, is impor- 2 tant in determining variation in δ H–CH4. The relationship 2 2 between δ H–CH4 and δ H–H2O leads to significant differ- 2 ences in the distribution of freshwater δ H–CH4 between the northern high latitudes (60–90◦ N), relative to other global Published by Copernicus Publications on behalf of the European Geosciences Union. 3506 P. M. J. Douglas et al.: Geographic variability in freshwater methane hydrogen isotope ratios 1 Introduction CH4 budgets (Kai et al., 2011; Rice et al., 2016), in part be- cause of loosely constrained source terms, as well as rela- Methane (CH4) is an important greenhouse gas that accounts tively sparse atmospheric measurements. Atmospheric inver- for approximately 25 % of current anthropogenic global sion models have shown that increased spatial and tempo- 2 warming, but we do not have a complete understanding of the ral resolution of δ H–CH4 measurements could provide sub- current relative or absolute fluxes of different CH4 sources stantial improvements in precision for global and regional to the atmosphere (Schwietzke et al., 2016; Saunois et al., methane budgets (Rigby et al., 2012). 2 2019), nor is there consensus on the causes of recent decadal- δ H–CH4 measurements could prove especially useful in 2 scale changes in the rate of increase in atmospheric CH4 (Kai understanding freshwater CH4 emissions. Freshwater δ H– 2 et al., 2011; Pison et al., 2013; Rice et al., 2016; Schaefer CH4 is thought to be highly dependent on δ H–H2O (Wal- et al., 2016; Worden et al., 2017; Thompson et al., 2018; dron et al., 1999a; Whiticar, 1999; Chanton et al., 2006). 2 Turner et al., 2019). Freshwater ecosystems are an integral Since δ H–H2O exhibits large geographic variation as a component of the global CH4 budget. They are one of the function of temperature and fractional precipitation (Rozan- 2 largest sources of atmospheric CH4 and are unequivocally ski et al., 1993; Bowen and Revenaugh, 2003), δ H–CH4 the largest natural, or non-anthropogenic, source (Bastviken measurements have the potential to differentiate freshwater et al., 2011; Saunois et al., 2019). At the same time the geo- CH4 sources by latitude. This approach has been applied in graphic distribution of freshwater CH4 emissions, changes in some ice core studies (Whiticar and Schaefer, 2007; Bock et the strength of this source through time, and the relative im- al., 2010), but geographic source signals remain poorly con- portance of wetland versus inland water CH4 emissions all strained, in part because of small datasets and because of in- 2 remain highly uncertain (Pison et al., 2013; Schaefer et al., completely understood relationships between δ H–H2O and 2 2016; Ganesan et al., 2018; Saunois et al., 2019; Turner et δ H–CH4. In contrast, recent studies of modern atmospheric 2 al., 2019). Gaining a better understanding of freshwater CH4 δ H–CH4 have typically not accounted for geographic vari- emissions on a global scale is of great importance for under- ation in freshwater CH4 sources (Kai et al., 2011; Rice et standing potential future climate feedbacks related to CH4 al., 2016). Relatedly, other studies have found an important 2 2 emissions from these ecosystems (Bastviken et al., 2011; role for variation in δ H–H2O in controlling δ H–CH4 from Koven et al., 2011; Yvon-Durocher et al., 2014; Zhang et biomass burning (Umezawa et al., 2011) and from plants ir- al., 2017). It is also necessary in order to better constrain the radiated by UV light (Vigano et al., 2010), as well as the 2 quantity and rate of change of other CH4 emissions sources, δ H of H2 produced by wood combustion (Röckmann et al., including anthropogenic sources from fossil fuels, agricul- 2016). 2 ture, and waste (Kai et al., 2011; Pison et al., 2013; Schaefer In addition to variance caused by δ H–H2O, a number et al., 2016). of additional biogeochemical variables have been proposed 13 2 Isotopic tracers, particularly δ C, have proven to be very to influence δ H–CH4 in freshwater environments. These useful in constraining global CH4 sources and sinks (Kai include differences in the predominant biochemical path- et al., 2011; Nisbet et al., 2016; Rice et al., 2016; Schae- way of methanogenesis (Whiticar et al., 1986; Whiticar, fer et al., 2016; Schwietzke et al., 2016; Nisbet et al., 2019). 1999; Chanton et al., 2006), the extent of methane oxida- However, δ13C source signatures cannot fully differentiate tion (Happell et al., 1994; Waldron et al., 1999a; Whiticar, CH4 sources, leaving residual ambiguity in source apportion- 1999; Cadieux et al., 2016), isotopic fractionation resulting ment (Schaefer et al., 2016; Schwietzke et al., 2016; Wor- from diffusive gas transport (Waldron et al., 1999a; Chanton, den et al., 2017; Turner et al., 2019). Applying additional 2005), and differences in the thermodynamic favorability or isotopic tracers to atmospheric CH4 monitoring has the po- enzymatic reversibility of methanogenesis (Valentine et al., tential to greatly improve our understanding of CH4 sources 2004b; Stolper et al., 2015; Douglas et al., 2016). These in- 2 and sinks (Turner et al., 2019; Saunois et al., 2020). Re- fluences on δ H–CH4 have the potential to complicate ge- cently developed laser-based methods, including cavity ring- ographic signals but also provide the potential to differen- down spectroscopy, quantum cascade laser absorption spec- tiate ecosystem sources, if specific ecosystems are charac- troscopy, and tunable infrared laser direct absorption spec- terized by differing rates and pathways of methanogenesis, troscopy, in addition to continued application of isotope ratio rates of CH4 oxidation, or gas transport processes. A recent 13 mass spectrometry (Chen et al., 2016; Röckmann et al., 2016; study proposed that freshwater δ C-CH4 could be differen- Yacovitch et al., 2020) could greatly enhance the practical- tiated geographically based on ecosystem differences in the 2 ity of atmospheric δ H–CH4 measurements at greater spatial prevalence of different methanogenic pathways and in the and temporal resolution, similar to recent developments for predominance of C4 plants, in addition to the geographic dis- 13 2 δ C-CH4 measurements (Zazzeri et al., 2015; Miles et al., tribution of wetland ecosystems (Ganesan et al., 2018).
Details
-
File Typepdf
-
Upload Time-
-
Content LanguagesEnglish
-
Upload UserAnonymous/Not logged-in
-
File Pages23 Page
-
File Size-