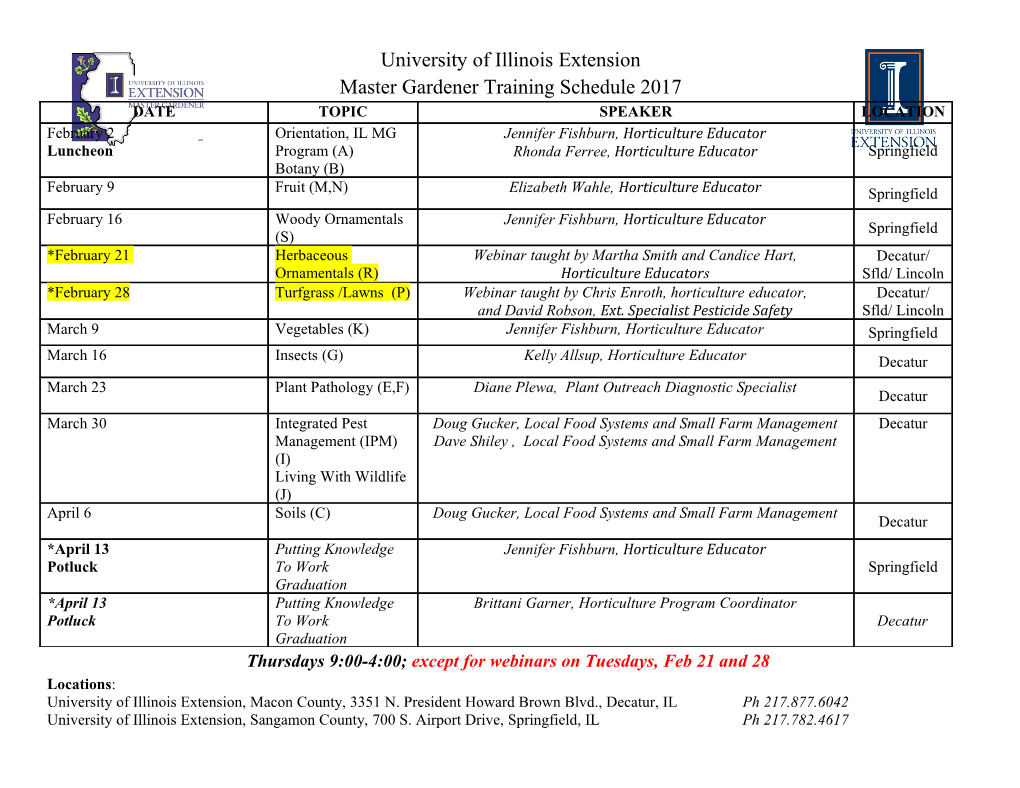
Selected for a Viewpoint in Physics week ending PRL 110, 130801 (2013) PHYSICAL REVIEW LETTERS 29 MARCH 2013 One-Particle Measurement of the Antiproton Magnetic Moment J. DiSciacca,1 M. Marshall,1 K. Marable,1 G. Gabrielse,1,* S. Ettenauer,1 E. Tardiff,1 R. Kalra,1 D. W. Fitzakerley,2 M. C. George,2 E. A. Hessels,2 C. H. Storry,2 M. Weel,2 D. Grzonka,3 W. Oelert,3,4 and T. Sefzick3 (ATRAP Collaboration) 1Department of Physics, Harvard University, Cambridge, Massachusetts 02138, USA 2Department of Physics and Astronomy, York University, Toronto, Ontario M3J 1P3, Canada 3IKP, Forschungszentrum Ju¨lich GmbH, 52425 Ju¨lich, Germany 4Institut fu¨r Physik, Johannes Gutenberg Universita¨t Mainz, D-5509 Mainz, Germany (Received 21 January 2013; published 25 March 2013) For the first time a single trapped antiproton (p) is used to measure the p magnetic moment p . The moment p ¼ p S=ð@=2Þ is given in terms of its spin S and the nuclear magneton (N)byp =N ¼ À2:792 845 Æ 0:000 012. The 4.4 parts per million (ppm) uncertainty is 680 times smaller than previously realized. Comparing to the proton moment measured using the same method and trap electrodes gives ¼1 000 000 Æ 0 000 005 ¼ S ð@ 2Þ p =p : : to 5 ppm, for a proton moment p p = = , consistent with the prediction of the CPT theorem. DOI: 10.1103/PhysRevLett.110.130801 PACS numbers: 14.20.Dh, 11.30.Er, 13.40.Em, 37.10.Ty Measurements of the properties of particles and antipar- This Letter reports the first single-particle measurement ticles are intriguing in part because the fundamental cause of the p magnetic moment, a 4.4 ppm determination that is of the asymmetry between matter and antimatter in the 680 times more precise than realized with exotic atoms universe has yet to be discovered. Within the standard (Fig. 1). The methods and apparatus were initially demon- model of particle physics, the results of particle- strated in a one-proton measurement of p [6], following antiparticle comparisons are predicted by a CPT theorem the realization of feedback cooling and a self-excited oscil- [1] that pertains because systems are described by a local, lator with one proton [7]. We profited from a parallel Lorentz-invariant, quantum field theory (QFT). Whether exploration of proton spin flips [8] and a measurement of the theorem applies universally is open to question, espe- p [9] that followed ours. cially since gravitational interactions have so far eluded a The cyclotron and spin frequencies (fc and fs), mea- QFT description. It is thus important to precisely test sured for a single p suspended in a magnetic field, deter- predictions of the CPT theorem, one example of which is mine the p moment in nuclear magnetons, that antiproton (p) and proton (p) magnetic moments have p g q =m g f opposite signs and the same magnitude. Testing this pre- p p p À p ¼ s ; (1) diction may eventually produce a second precise CPT test N 2 qp=mp 2 fc with a baryon and antibaryon, of comparable precision to where g is the pg factor. The ratio of p and p charge-to- the p and p charge-to-mass ratio comparison [2]. p The p magnetic moment was previously deduced only mass ratios enters because the nuclear magneton N is defined in terms of the proton charge and mass. This ratio from measured transition energies in exotic atoms in which À1 a p orbits a nucleus as a ‘‘heavy electron.’’ Measurements was measured to be to 0.0001 ppm using a p 25 and 4 years ago [3,4] both reached a 3000 ppm precision (Fig. 1). Meanwhile, single particle methods were used to measure other magnetic moments to a much higher preci- sion. For example, the most precisely measured property of an elementary particle is the electron magnetic moment measured with one electron [5]. Published by the American Physical Society under the terms of the Creative Commons Attribution 3.0 License. Further distri- bution of this work must maintain attribution to the author(s) and FIG. 1. Uncertainties in measurements of the p magnetic mo- the published article’s title, journal citation, and DOI. ment measured in nuclear magnetons, p =N. 0031-9007=13=110(13)=130801(5) 130801-1 Published by the American Physical Society week ending PRL 110, 130801 (2013) PHYSICAL REVIEW LETTERS 29 MARCH 2013 À simultaneously trapped with a H ion [2] so the approxi- frequency fÀ ¼ 5:32 kHz. The spin precession frequency mation in Eq. (1) is more than adequate for our precision. is fs ¼ 221:075 MHz. The p magnetic moment is measured within the ‘‘analy- Driving spin flips requires a magnetic field perpendicu- sis trap’’ electrodes (Fig. 2) used to measure the proton lar to B that oscillates at approximately fs. This field is magnetic moment [6]. The stacked rings are made of OFE generated by currents (increased compared to Ref. [6]bya copper or iron, with a 3 mm inner diameter and an evapo- transmission line transformer) sent through halves of a rated gold layer. The electrodes and surrounding vacuum compensation electrode [Fig. 2(c)]. Driving cyclotron container are cooled to 4.2 K by a thermal connection to transitions requires an electric field perpendicular to B liquid helium. Cryopumping of the closed system made the that oscillates at approximately fþ. This field is generated vacuum better than 5  10À17 Torr in a similar system by potentials applied across halves of a compensation [10], so collisions are unimportant. Appropriate potentials electrode [Fig. 2(d)]. applied to electrodes with a carefully chosen relative ge- Much of the challenge of the measurement arises from ometry [11] make a very good electrostatic quadrupole the small size of a nuclear magnetic moment. Unlike the near the trap center with open access to the trap interior electron moment, which scales naturally as a Bohr mag- from either end. neton (B), the nuclear moments scale as the much smaller After the proton measurement [6] was completed, the nuclear magneton N, with N=B ¼ me=mp 1=2000. apparatus was modified and moved from Harvard to Shifts in fz reveal changes in the cyclotron, spin, and CERN. The neighboring electrodes and vacuum enclosure magnetron quantum numbers n, ms, and ‘ [13], (not pictured in Fig. 2) were modified to allow 5 MeV p Á @ 1 g m 1 from CERN’s antiproton decelerator (AD) to enter the fz ¼ 2 þ þ p s þ fÀ þ 4 j j n 2 2 ‘ 2 : vacuum enclosure through a thin Ti window and to be fz mp B fÀ fþ captured and electron cooled in the neighboring electrodes. (2) The cooling electrons are ejected by reducing the trap potential long enough that light electrons escape while The shifts (50 and 130 mHz per cyclotron quantum and heavier p do not. These methods, now used for all low spin flip) arise from a saturated iron ring [Fig. 2(a)] that B energy p and H experiments, are reviewed in Ref. [12]. adds (to ) a magnetic bottle gradient (at the trap center), Once the p is centered in the analysis trap, in a 5 tesla 2 2 ÁB ¼ 2½ðz À =2Þz^ À z^: (3) vertical magnetic field B ¼ Bz^, the circular cyclotron motion of a trapped p is perpendicular to B with a fre- The effective fz shifts because the electrostatic axial oscil- 2 2 2 quency fþ ¼ 79:152 MHz slightly shifted from fc by the lator Hamiltonian going as fz z acquires z from the electrostatic potential. The p also oscillates parallel to B at interaction of the cyclotron, magnetron, and spin moments, ^ 5 2 about fz ¼ 920 kHz. The third motion is a circular mag- z, with ÁB. The bottle strength, 2 ¼ 2:9  10 T=m , netron motion, also perpendicular to B, at the much lower is 190 times that used to detect electron spin flips [5]to compensate partially for the small N. The p are transferred between the analysis trap and an adjacent coaxial trap (not in Fig. 2) by slowly varying the (a) 3 m m (b) B (tesla) - 5.67 applied electrode voltages to make the axial potential well move adiabatically between the two trap centers. In the end c ap adjacent trap the p cyclotron motion induces currents in c o m p ens at i o n and comes to thermal equilibrium with an attached damp- m ) iro n ring ing circuit cooled with the trap. The cooled p is transferred Á c o m p ens at i o n back to the analysis trap and a measured shift fz < 100 Hz is required to ensure a cyclotron radius below distanc e distanc ( end c ap 0:7 m (a bit larger than was possible with more time in Ref. [6]) before measuring fs. For larger shifts, the p is returned to the precision trap for cyclotron damping as fc drive fs drive needed until a low cyclotron energy is selected. Two methods are used to measure the Áfz of Eq. (2)in the analysis trap, though the choice of which method to use (c) (d) in which context is more historical than necessary at the lower compensation lower compensation current precision. The first (used to detect cyclotron tran- Á FIG. 2 (color online). (a) Electrodes of the analysis trap (cut- sitions with the weakest possible driving force) takes fz away side view) are copper with an iron ring. (b) The iron ring to be the shift of the frequency at which Johnson noise in a significantly alters B on axis.
Details
-
File Typepdf
-
Upload Time-
-
Content LanguagesEnglish
-
Upload UserAnonymous/Not logged-in
-
File Pages5 Page
-
File Size-