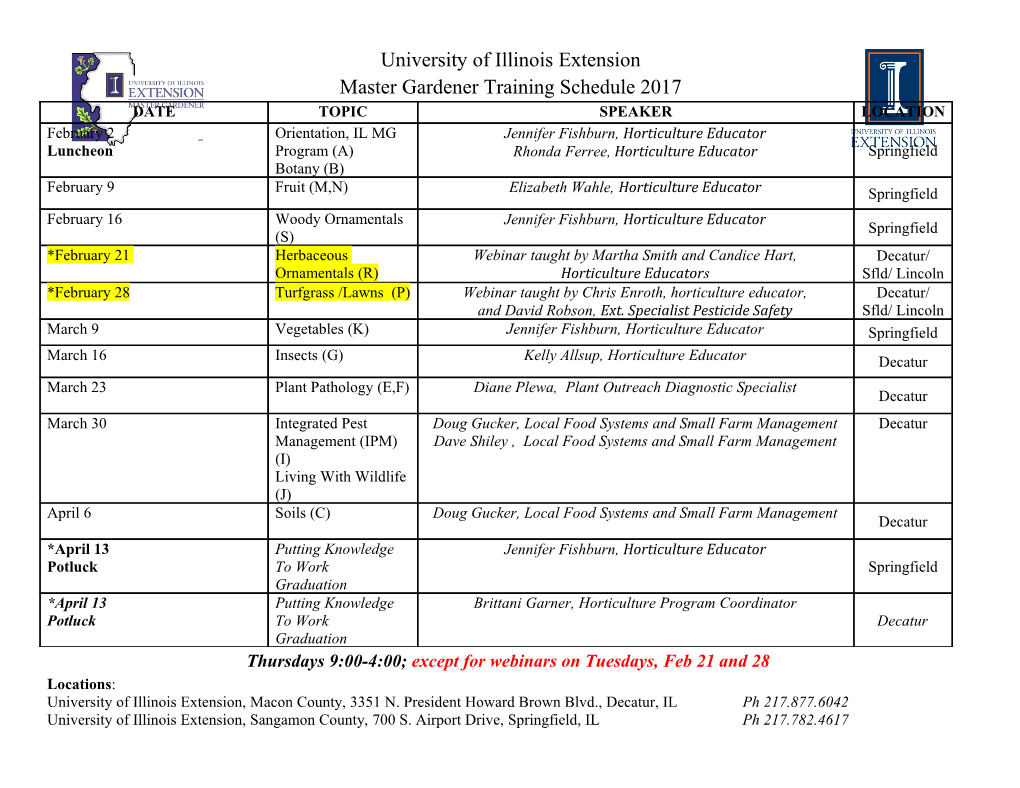
56 Distributed Generation and Alternative Energy Journal Addressing Solar-PV Power Generation: Commercialization Assessment for the US Energy Market Mahdi Yaqub San Jose State University Shahram Sarkani Thomas A. Mazzuchi George Washington University ABSTRACT Solar-Photovoltaic (PV) utility power is more expensive to produce compared with conventional sources. Current utility power purchase agreement policies discourage private investment because far future cash flow does not add to asset value. This article presents an overview of a study that assesses the commercialization of PV power generation in the US energy market. Data analysis substantiates that for PV power to be competitive with conventional power plants, much lower discount rates are required during the first half of the PV utility lifecycle, after which solar PV will have a much lower cost due to a drastic reduction in the cost of capital. Additionally, the levelized cost of energy analysis for a longer lifecycle indicates that the utility scale solar PV cost gap can be bridged. Therefore, this article aims to influence policy makers to in- troduce long-term power purchase agreements, taking into account the avoided costs due to the unevaluated quality of long life at anticipated low operating costs. Furthermore, simulations reveal that the proposed solar PV self-financing program may be a viable alternative to the cur- rent government subsidy that lacks an inflow of cash to offset the out- flow of subsidy payments. Finally, we present selective strategies that can help drive the commercialization of PV power generation in the US energy market. Key words: levelized cost of energy (LCOE), solar-photovoltaic (PV), investment incentives, technology commercialization, smart grid, commercialization barriers and drivers, technology adoption. Vol. 28, No. 1 2013 57 INTRODUCTION There is recent interest in utility-scale PV electricity generation for various reasons such as the increasing volatility of fossil fuel prices, the desire to reduce carbon emissions, and the availability of new technolo- gies, which make utility-scale solar PV electric power more attractive. However, a primary hurdle to the widespread deployment of PV electric utilities is that PV electricity is more expensive to produce than com- peting energy sources such as coal and natural gas, which overlooks the vital PV solar system long-life characteristic with anticipated low operating costs. Moreover, the power generation sector is undergoing increasingly stringent environmental regulations, a strong policy push for aggressive emission reduction, volatile fossil fuel prices, new elec- tricity transmission modernization (smart grid), and new entry of less expensive cadmium telluride (CdTe) solar PV technology. Figure 1. Organizational Flow of this Article’s Presentation 58 Distributed Generation and Alternative Energy Journal These new energy market conditions can make future coal and natural gas power plants less competitive with alternative distributed generation power sources such as solar PV. In response to these new energy market realities, we redefine the levelized cost of energy (LCOE) and present two mathematical formulas. The first represents the inves- tors’ viewpoint and allows for transmission and access and upgrade costs and accounts for degradation over the project’s lifecycle. The sec- ond represents the societal economic value viewpoint and accounts for carbon cost, hedging, peak supply, transmission loss, and reserve costs. Additionally, we propose and simulate a finance and investment pro- gram to be considered as a viable alternative to the current government subsidy that lacks an inflow of cash to offset the outflow of subsidy pay- ments. The intent of the proposed model is to begin discussion around the self-financing program and the role of public policy, as well as to use simulations to facilitate strategic planning and decision making. We uti- lize system dynamics methodology to investigate PV power long-term economic outlooks. Such methods are introduced by system dynamics pioneers, namely J. W. Forrester [7] and John Sterman [8]. Finally, this article suggests a five-parts plan to help commercialize PV technology in the US energy market. Figure 1 represents the organizational flow of this article’s presentation. PV TECHNOLOGY BARRIERS AND DRIVERS The US energy market still has a high carbon footprint because of electricity generation. For example, US commercial and industrial en- ergy combined account for 50% of U.S. energy consumption. Fossil fuels are still the dominant energy source (approximately 85%); the top three are coal, natural gas, and oil [1]. Other energy sources are nuclear, wind, solar, biomass, and wave/tidal. Figure 2 illustrates US electricity genera- tion by source. Current US energy market risks are resource depletion, supply production peaks, electric supply’s security, environmental impact, and renewable energy cost. Thus, we need an energy policy approach to as- sess the feasibility of renewable energy technology infusion. This study focuses on the feasibility of solar PV technology infusion in the national electric power system to achieve a low carbon economy. Table 1 summa- rizes the key barriers and drivers of PV power commercialization. Vol. 28, No. 1 2013 59 Figure 2. US Electricity Generation by Source Table 1. Solar PV Power Generation Key Barriers and Drivers PV TECHNOLOGY COST COMPETITIVENESS Currently, power purchase agreements (PPAs) are 8c-9c/kWh and are difficult to finance even at lower module prices (50% of project cost) without subsidies. To assess if solar-PV (or PV for short) technology is cost competitive in the long run; we evaluate the following cost parametric in- fluence implications. We constructed Table 2 based on data extracted from “Updated Capital Cost Estimates for Electricity Generation Plants” [2] 60 Distributed Generation and Alternative Energy Journal and the National Renewable Energy Laboratory (NREL) System Advisor Model [3]. Table 2 compares the solar PV present value of annual energy cost to the two main energy sources: coal (constitutes 50 percent of total US electricity generation) and natural gas (constitutes 35 percent of total US electricity generation). We calculated the annual costs’ present value based on the 30-year project lifecycle without government subsidy. Table 2 demonstrates that solar PV has the highest present value cost of energy compared with coal and natural gas power plants. Table 2. Utility Sources Annual Cost Comparison ($/MWh) Currently, Levelized Cost of Energy (LCOE) calculations lack clarity and completeness, leading to widely varying, inconsistent, and conflicting results. A literature search reveals that most studies do not consider the energy output degradation that leads to the reduction in energy production. Many widespread LCOE calculations do not express such a cost parameter. The following investors’ economic value method attempts to capture such an important cost factor. LCOE Investors’ Economic Value Method The LCOE is a metric utilized to assess the all-in-one unit cost of generating utility electric power from different energy sources. It includes key project expenses such as the cost of capital, operation and maintenance (O&M) costs, and debt. It is expressed in dollars per megawatt-hours ($/MWh) or cents per kilowatt-hours (cents/KWh) [4]. To calculate the levelized cost of a power plant, we converted expenses to annualized payments during the project life. The annualized cost is then divided by the average annual generation (MWh). There are two key components of the levelized cost: fixed costs and variable costs. The costs of capital payments and fixed O&M costs are components of the fixed costs. Variable costs include variable O&M expenses and fuel costs. For utility projects, we calculate the LCOE such that when the LCOE is multiplied by the utility lifecycle total produced energy and discounted to the assumed analysis year, it can then be represented by the utility project’s lifecycle present value of required revenues. In the Vol. 28, No. 1 2013 61 following equation, we represent the LCOE in a simplified mathematical form. Equation 1 is derived from the Department of Energy’s general- ized function to generate the annual net present value cost of energy; however, we add a “df” degradation factor variable to represent the reduction in energy output due to system-wide tear and wear over time. Thus, the LCOE function can be expressed as N R required,n × PV LCOE = Σn=1 Qn (1 – df) n (1+d) (1) In equation (1), Qn is the energy produced (MWh) by the project in year n. N is the project life in years, and “Required,n” is the required project revenue resulting from electricity generation in year n ($/MWh), df is the degradation factor, and d is the discount-rate. The right hand summation starts at n = 1 (the first year of project revenues). To better understand the competitiveness of the PV LCOE, we evaluate the fol- lowing PV technology cost influences. Solar PV Exceptionally High Cost of Capital Implication Table 3 indicates that the levelized cost of capital of coal power plants is approximately 59% compared with 28% for natural gas and 95% compared with PV power plants [2, 11], as a fraction of their total PV LCOE. Hence, solar PV cost of capital is the key variable metric with 95% share of the total cost, as Figure 3 illustrates. Accordingly, discount rates have a particularly significant impact on solar PV cost competitiveness. PPA Lifecycle Implication We evaluate solar PV LCOE over a longer lifecycle of 60 years, shedding light on solar PV’s low operating cost and long life advan- tages. We employ Minitab statistical software to calculate and plot the solar PV LCOE weighted average based on the data extracted from the Table 3. Estimated Percentage of LCOE for New Power Plant Construction 62 Distributed Generation and Alternative Energy Journal Figure 3. Coal, Natural Gas, and PV Utilities Cost of Capital Comparison National Renewable Energy Laboratory, Energy Analysis, and System Advisory Model (SAM). Figure 4 plots the weighted average LCOE for the first 18 years and again for the remainder of the 60-year lifecycle.
Details
-
File Typepdf
-
Upload Time-
-
Content LanguagesEnglish
-
Upload UserAnonymous/Not logged-in
-
File Pages25 Page
-
File Size-