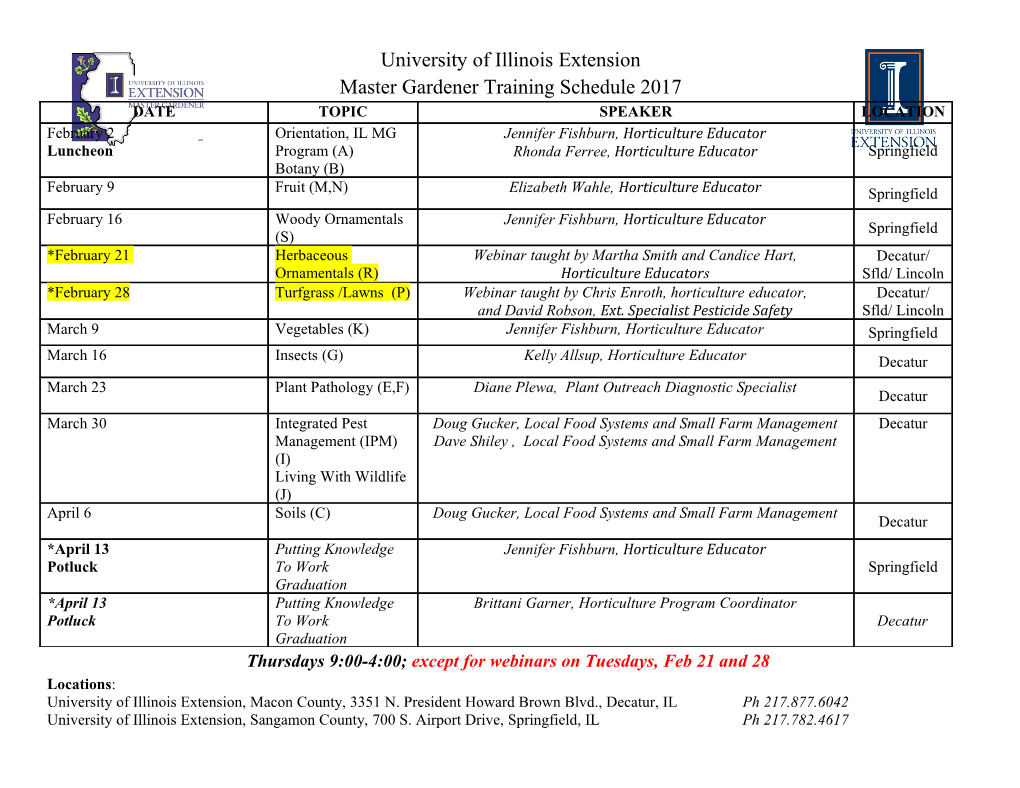
University of California Los Angeles Study of Flow, Turbulence and Transport on the Large Plasma Device A dissertation submitted in partial satisfaction of the requirements for the degree Doctor of Philosophy in Physics by David Andrew Schaffner 2013 c Copyright by David Andrew Schaffner 2013 Abstract of the Dissertation Study of Flow, Turbulence and Transport on the Large Plasma Device by David Andrew Schaffner Doctor of Philosophy in Physics University of California, Los Angeles, 2013 Professor Troy A. Carter, Chair The relationships amongst azimuthal flow, radial particle transport and turbulence on the Large Plasma Device (LAPD) are explored through the use of biasable limiters which con- tinuously modify the rotation of the plasma column. Four quarter annulus plates serve as an iris-like boundary between the cathode source and the main plasma chamber. Application of a voltage to the plates using a capacitor bank drives cross-field current which rotates the plasma azimuthally in the electron diamagnetic direction (EDD). With the limiters inserted, a spontaneous rotation in the ion diamagnetic direction is observed; thus, increasing biasing tends to first slow rotation, null it out, then reverse it. This experiment builds on previous LAPD biasing experiments which used the chamber wall as the biasing electrode rather than inserted limiter plates. The use of inserted limiter biasing rather than chamber wall biasing allows for better cross-field current penetration between the plasma source and the electrodes which in turn allow for a finer variation of applied torque on the plasma. The modification of plasma parameter profiles, turbulent characteristics, and radial trans- port are tracked through these varying flow states. Azimuthal flow radial profiles are peaked at the limiter edge. Consequently, the variation in flow states also results in variation of sheared flow. Improved radial particle confinement is observed in states with sheared flow regardless of the direction of rotation. This improvement is indicated by both steepened den- sity profiles and decreased radial particle flux. Conversely, a confinement degradation is seen ii in the minimum sheared flow state. Comparison of density fluctuation power and crossphase between density and radial velocity fluctuations show that both quantities are suppressed by sheared flow, but that the density fluctuation suppression is dominant and contributes most to the decrease in radial particle flux. Also, some observed changes to density and flux profiles suggest disagreement with a purely local model of transport including the formation of a hollow density profile at state of high flow (high bias) and regions where the direction of measured flux is opposite to that predicted by the direction of density gradient. Changes in flux and density gradient with bias are correlated but in a way that is inconsistent with a Fick's Law like model. Changes in turbulent spectra and features are observed with modification of the rotation state. Log-linear spectra with rotation in either azimuthal direction tends to exhibit a linear slope for power versus frequency and versus wavenumber, while spectra curves in the null flow case have an upward concave shape. Time traces corresponding to these spectra indicate a clear presence of Lorentzian structures in flow states but not in the null flow state. Spectral density histograms, showing the distribution of density fluctuation power in both frequency and wavenumber space, indicate that the modes tend to propagate azimuthally in the same direction as the flow. This suggests a large contribution of rotational interchange instability to the plasma turbulence. Regions with significant flow exhibit spectral density distributions which follow narrow curves in frequency and wavenumber space, much like a dispersion relation line. Regions without flow (either the null flow state or the plasma core) have broader, more diffuse distributions. A pair of coherent modes develop with rotation in the EDD direction. The mode fluctua- tion power peaks at the limiter edge; since maximum flow is observed at the limiter edge, the modes are most likely flow driven. Comparison with a linear Braginskii-fluid model eigen- function solver supports this conclusion. The lower frequency mode—first observed at about 1-2kHz|is identified as a pure interchange mode while the higher frequency mode—first observed at about 5-6kHz|is determined to be a coupled drift-wave interchange mode. The frequency of these modes increases with flow velocity; the appearance of sideband modes in iii the frequency spectra also suggests that these mode begin to interact. iv The dissertation of David Andrew Schaffner is approved. Jon Aurnou George Morales Walter Gekelman Troy A. Carter, Committee Chair University of California, Los Angeles 2013 v To Erin, of course. And to my parents for setting me down this path. vi Table of Contents 1 Overview of the Study of Flow, Turbulence, and Transport on the Large Plasma Device :::::::::::::::::::::::::::::::::::::: 1 1.1 Control of Plasma Rotation on LAPD......................1 1.2 Modification of Density Profile and Radial Particle Confinement.......3 1.3 Modification of Turbulence............................5 1.4 Emergence of a Coherent Mode.........................6 1.5 Local Analysis of Turbulence and Transport variation with shearing rate..8 1.6 Summary of Dissertation Chapters.......................8 2 Introduction and Motivation ::::::::::::::::::::::::::: 11 2.1 From H-Mode to Biasing Experiments..................... 12 2.2 Turbulence, Transport and Shear........................ 16 2.2.1 The Role of Rotation Experiments on LAPD.............. 16 2.3 Plasma Flows................................... 17 2.3.1 ExB Drift................................. 17 2.3.2 General Force Drift and Diamagnetic Drift............... 20 2.4 Diffusion: Classical and Bohm.......................... 21 2.4.1 Parallel Diffusion............................. 25 2.4.2 Perpendicular Diffusion.......................... 26 2.4.3 Anomalous Diffusion........................... 28 2.5 Relevant Instabilities on a Linear Device.................... 29 2.5.1 Resistive Drift Wave Instability..................... 29 2.5.2 Rotational Interchange Instability.................... 31 vii 2.5.3 Kelvin-Helmholtz............................. 31 3 LAPD and Diagnostics ::::::::::::::::::::::::::::::: 33 3.1 The Large Plasma Device............................ 33 3.1.1 Axial versus Radial Transport...................... 39 3.2 Diagnostics.................................... 41 3.2.1 Langmuir Probes............................. 41 3.2.2 Swept Probes............................... 42 3.2.3 Mach Probe................................ 43 3.2.4 Nine-Tip Langmuir Probe........................ 48 3.2.4.1 Density............................. 49 3.2.4.2 Floating Potential and Local Electric Field fluctuations... 49 3.2.4.3 Particle Flux, Reynolds Stress and Vorticity......... 50 3.2.5 Data Acquisition Settings........................ 51 3.2.5.1 Probe Axial Positions..................... 51 3.2.6 Fast-Framing Camera........................... 52 4 Bias-driven plasma rotation :::::::::::::::::::::::::::: 54 4.1 The Limiters................................... 54 4.2 The Biasing Circuit................................ 55 4.3 Limiter Voltage and Discharge Current Response with Bias.......... 61 4.3.1 Field Variations.............................. 66 4.4 Modification of Plasma due to the presence of Limiter Plates......... 68 4.5 Setting Plasma Potential, Flow and Shear with Biasing............ 72 4.6 Cross-field Current and Pedersen Conductivity................. 82 viii 5 Modification of LAPD turbulence with steady-state biased rotation ::: 87 5.1 Profile Modification................................ 87 5.1.1 Density and Temperature Profiles.................... 87 5.1.2 Density, Potential and Velocity Fluctuation Profiles.......... 95 5.1.3 Particle Flux and Diffusivity Profiles.................. 100 5.2 Spectrum Modification.............................. 107 5.2.1 Frequency Spectral Distribution..................... 109 5.2.2 Turbulent Spatial Distribution...................... 119 5.2.3 Wavenumber and M-Number Spectra.................. 122 5.3 Mode Analysis.................................. 129 5.3.1 Instability Drive.............................. 131 5.4 Fluctuation Ratios................................ 133 5.5 Linear Analysis Fluid Equations......................... 134 5.5.1 Experimental Spectra vs Linear Growth................ 140 5.5.2 Evaluation of the Coherent Mode.................... 149 5.6 Turbulent Structure and Modification...................... 160 6 Local analysis of the variation of turbulence and transport with shearing rate ::::::::::::::::::::::::::::::::::::::::::::: 167 6.1 Flow and Density................................. 167 6.2 Decorrelation Rate................................ 170 6.3 Density Gradient Modification.......................... 172 6.4 Turbulence and Transport Suppression..................... 174 6.4.1 Density Fluctuations........................... 177 6.4.2 Crossphase................................ 180 ix 6.4.3 Radial Velocity Fluctuations....................... 182 6.4.4 Coherence................................. 182 6.5 Radial Correlation Length............................ 186 7 Quantitative Scaling of turbulent quantities with sheared flow :::::: 189 7.1 Models of Shear Suppression........................... 189 7.2 Experimental Shear Suppression Scaling.................... 191 7.2.1 Density and Velocity Fluctuations.................... 200
Details
-
File Typepdf
-
Upload Time-
-
Content LanguagesEnglish
-
Upload UserAnonymous/Not logged-in
-
File Pages281 Page
-
File Size-