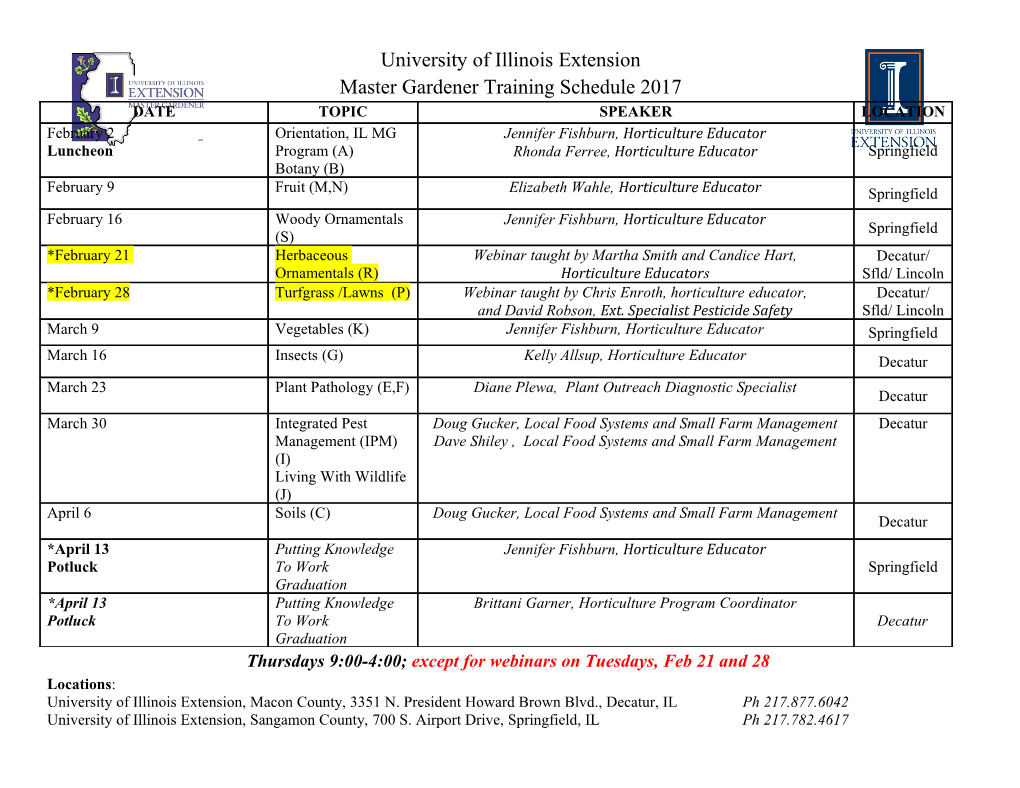
Developments in Earth & Environmental Sciences, 8 F. Florindo and M. Siegert (Editors) r 2009 Elsevier B.V. All rights reserved DOI 10.1016/S1571-9197(08)00009-8 1 Chapter 9 3 5 The Oligocene–Miocene Boundary – 7 Antarctic Climate Response to Orbital Forcing 9 1,Ã 2 3 4 11 G. S. Wilson , S. F. Pekar , T. Naish , S. Passchier and R. DeConto5 13 1Department of Geology, University of Otago, P.O. Box 56, Dunedin, New Zealand 2School of Earth and Environmental Sciences, Queens College, CUNY, 65-30 Kissena 15 Blvd., Flushing, NY 11367, USA and Lamont Doherty Earth Observatory of Columbia University, Palisades, NY 10964, USA 17 3Antarctic Research Centre, Victoria University, P.O. Box 600, Wellington, New Zealand and GNS Science, P.O. Box 30368, Lower Hutt, New Zealand 19 4Department of Earth and Environmental Studies, Montclair State University, 252 Mallory Hall, 1 Normal Avenue, Montclair, NJ 07403, USA 21 5Department of Geosciences, University of Massachusetts, 233 Morril Science Center, 611 North Pleasant Street, Amherst, MA 01003-9297, USA 23 25 ABSTRACT 27 Recent high-resolution Oligocene–Miocene oxygen isotopic records revealed a AU :2 relatively transient, ca. 2 myr period, 1 m amplitude cyclicity in isotopic values (Oi and Mi events, respectively). Intriguingly, it has been suggested that these isotopic 29 excursions in oceanic d18O were linked to ephemeral growth and decay in Antarctic ice sheets. A great deal of effort in the palaeoceanography community 31 has been focused on developing techniques and gathering further records to determine if the Antarctic ice has behaved in such a transient manner in the past 33 and indeed what factors might have led to the rapid growth and decay of ice sheets. Deciphering between temperature and ice-volume influences in the deep-sea 35 isotopic record has proven somewhat difficult. Approaches have included the sampling of sediment from beneath different water masses, development of an 37 independent palaeothermometer using magnesium/calcium ratios and improving the resolution and accuracy of coastal sea-level records. Despite these advances it 39 ÃCorresponding author. 41 E-mail: [email protected] (G.S. Wilson). 374 G. S. Wilson et al. 1 is only through the recovery of Antarctic drill core records that we have been able to test the resulting hypotheses. Combined with numerical climate models, 3 ice-volume estimates are also available.The Antarctic Oligocene–Miocene record is most complete in the Victoria Land Basin as recovered in the CIROS-1 and 5 CRP-2A drill holes. The strata recovered in both drill holes are cyclic in nature and interpreted to represent periodic advance and retreat of ice across the Antarctic margin concomitant with sea-level fall and rise, respectively. While, 7 environmental data suggest a significant Antarctic climate threshold across the Oligocene–Miocene boundary with cooler temperatures implied in the early 9 Miocene, ice volume and palaeo-sea-level estimates suggest a significant but transient growth in the Antarctic ice sheet to B25% larger than present. The 11 Antarctic data are entirely consistent with the predictions from deep-sea records including the suggestion that the glacial advance was relatively short lived and 13 interglacial conditions were re-established within a few hundred thousand years.The duration and transience of the Mi1 glacial expansion and swift 15 recovery in Antarctica likely resulted from the limited polar summer warmth from the coincidence of low eccentricity and low-amplitude variability in obliquity of the 17 earth’s orbit at the Oligocene–Miocene boundary. This was followed by warmer polar summers and increased melt from increased eccentricity and high-amplitude variability in obliquity in the early Miocene, allowing the recovery of vegetation on 19 the craton. Atmospheric CO2 concentrations remained below a 2 Â pre-industrial threshold, which promoted sensitivity of the climate system to orbital forcing. 21 While climate and ice-sheet modelling support the fundamental role of greenhouse gas forcing as a likely cause of events like Mi1, the models underestimate the 23 range of orbitally paced ice-sheet variability recognised in early Miocene isotope and sea-level records unless accompanied by significant fluctuations in greenhouse 25 gas concentrations. While tectonic influence may have been secondary, they may well have contributed to oceanic cooling recorded at the Cape Roberts Project site 27 in the South Western Ross Sea. 29 31 9.1. Introduction 33 The paucity of Cenozoic outcrop on the Antarctic craton has led to the 35 reliance on proxy records (isotopic signatures in microfossils, deep-sea erosion events and former sea levels on distal continental margins) to help 37 unravel the history of climate and ice sheets on Antarctica (Kennett and Shackleton, 1976; Kennett, 1977; Wright and Miller, 1993; Miller and 39 Mountain, 1996; Miller et al., 2005; Zachos et al., 2001a). Much attention has been focused in the search for Antarctic data sets to ‘ground truth’ 41 significant climate trends, events and thresholds observed in these proxy The Oligocene–Miocene Boundary 375 1 records. Drilling around the Antarctic margin by seven legs of the Deep Sea Drilling Project (DSDP; Kennett et al., 1974; Hayes and Frakes, 1975) and 3 Ocean Drilling Programme (ODP; Barker et al., 1988a, b; Ciesielski et al., 1988; Barron et al., 1989; Barker et al., 1999; O’Brien et al., 2001) and several 5 sea-ice-based drilling projects (Barrett, 1986, 1989; Cape Roberts Science Team, 1998, 1999, 2000; Fig. 9.1) has recovered Cenozoic sequences, which 7 have allowed the testing of interpretations of Antarctic Glacial history from proxy records and climate models. While the DSDP and ODP core recovery 9 has been between 14 and 40%, riser drilling from sea ice in the South Western Ross Sea has enabled recovered of some high-quality intervals of 11 the Cenozoic (95–98%) recovering prima facie documentation of climate and cryospheric changes in Antarctica. One interval that is particularly well- 13 sampled and well-dated in several drill cores is the Oligocene–Miocene boundary, permitting an accurate comparison to deep-sea high-resolution 15 isotopic records from lower latitudes has been possible (Naish et al., 2001, 2008; Wilson et al., 2002; Roberts et al., 2003). 17 This chapter reviews recent evidence for a glacial expansion in Antarctica coincident with the Oligocene–Miocene boundary and the Mi1 deep-sea 19 oxygen isotope excursion. The climatic significance of the boundary has only recently become apparent from recalibration of the Oligocene–Miocene time 21 scale using astrochronology (Zachos et al., 2001b). Consequently, age data and chronstratigraphy of the Oligocene–Miocene boundary and the Antarctic 23 strata that contain the boundary are also reviewed. Data sets considered to indicate climate and ice-sheet variability across the Boundary include benthic 25 and planktic oxygen isotope (d18O) records (Kennett and Shackleton, 1976; Miller et al., 1991; Wright and Miller, 1992; Paul et al., 2000; Zachos et al., 27 2001b; Billups et al., 2002) and microfossil geochemistry (Billups and Schrag, 2002; Lear et al., 2004), sequence stratigraphic analyses of Antarctic (Fielding 29 et al., 1997; Naish et al., 2001, 2008) and mid-latitude (Kominz and Pekar, 2001; Pekar et al., 2002; Miller et al., 2005, 2006; Pekar and DeConto, 2006) AU :3 31 continental margin strata, Antarctic palaeobotany and palynology (Askin and Raine, 2000; Barrett, 2007) and physical properties of Antarctic drill core 33 strata including lithology, clay mineralogy, mudrock geochemistry and magnetic mineralogy (Verosub et al., 2000; Ehrmann et al., 2005; Passchier 35 and Krissek, 2008). Finally, the cause of the Mi1 glaciation is considered. 37 9.1.1. Identification of the Oligocene–Miocene Boundary 39 Early definition of the Oligocene–Miocene boundary relied on the identifica- 41 tion of the last occurrence of the calcareous nannofossil Dictyococcites 376 G. S. Wilson et al. ° 1 -30 3 929 CR 5 522 0° ° CEAN 30° 7 O SOUTH -60 TIC AFRICA SOUTH AN TL 9 AMERICA A 1090 60 ° I 11 N 689 D I KGI 690 MR A BS N 13 693 WS KP O DP C AP PB 747 E SSI A ° 748 15 EAIS 90 N ° -90 WAIS ° 80 ° WL 17 70 270 ° 60 RS ° 50 19 ° 40 80˚ ° 120 30 P ° A CIROS-1 21 ° C 20 IF I CRP-2a ° C IA 10 O VLB CE STRAL 23 AN AU ° RS -120 25 27 70 1218 ° 29 150 ° ° -150 31 DSDP/ODP/Antarctic Drillsites Mid Ocean Spreading Ridge 180° 33 Figure 9.1: Polar Stereographic Projection Showing the Location of Key 35 Oligocene–Miocene Boundary Sections Discussed in the Text. Abbreviations of Locations: Numbers are DSDP/ODP Drill sites; AP, Antarctic Peninsula; 37 BS, Bransfield Strait; CIROS, Cenozoic Investigations in the Ross Sea Drilling Project; CR, Ceara Rise; CRP, Cape Roberts Drilling Project; DP, 39 Drake Passage; EAIS, East Antarctic Ice Sheet; KGI, King George Island; KP, Kerguelen Plateau; MR, Maud Rise; PB, Prydz Bay; RS, Ross Sea; SSI, 41 South Shetland Islands; VLB, Victoria Land Basin; WAIS, West Antarctic Ice Sheet; WS, Weddell Sea. The Oligocene–Miocene Boundary 377 25 24 23 1 Age (Ma) Ness et al. (1980) C6Cn.3n C6Cn.2n C6Cr C6Cn.2r C6Cn.1r C6Cn.1n C6Br C6Bn 3 24.6 Ma 5 25 24 23 Age (Ma) 7 Berggren et al. (1985) C6Cn.1r C6Cr C6Cn.3n C6Cn.2r C6Cn.2n C6Cn.1n C6Br 9 23.74 Ma 11 25 24 23 Age (Ma) 13 Cande & Kent (1992, 1995) C6Cn.1r C6Cn.3n C6Cn.2n C7n.1n C6Cr C6Cn.2r C6Cn.1n C6Br 15 23.8 Ma 17 23 24 Age (Ma) Billups et al. (2004) C6Cn.1r C6Cn.3n C6Cn.2n 19 C7n.1n C6Cr C6Cn.2r C6Cn.1n C6Br 23.033 Ma 21 OLIGOCENE MIOCENE 23 Figure 9.2: History of the Calibration of the Geomagnetic Polarity Time Scale in the Vicinity of the Oligocene–Miocene Boundary.
Details
-
File Typepdf
-
Upload Time-
-
Content LanguagesEnglish
-
Upload UserAnonymous/Not logged-in
-
File Pages34 Page
-
File Size-