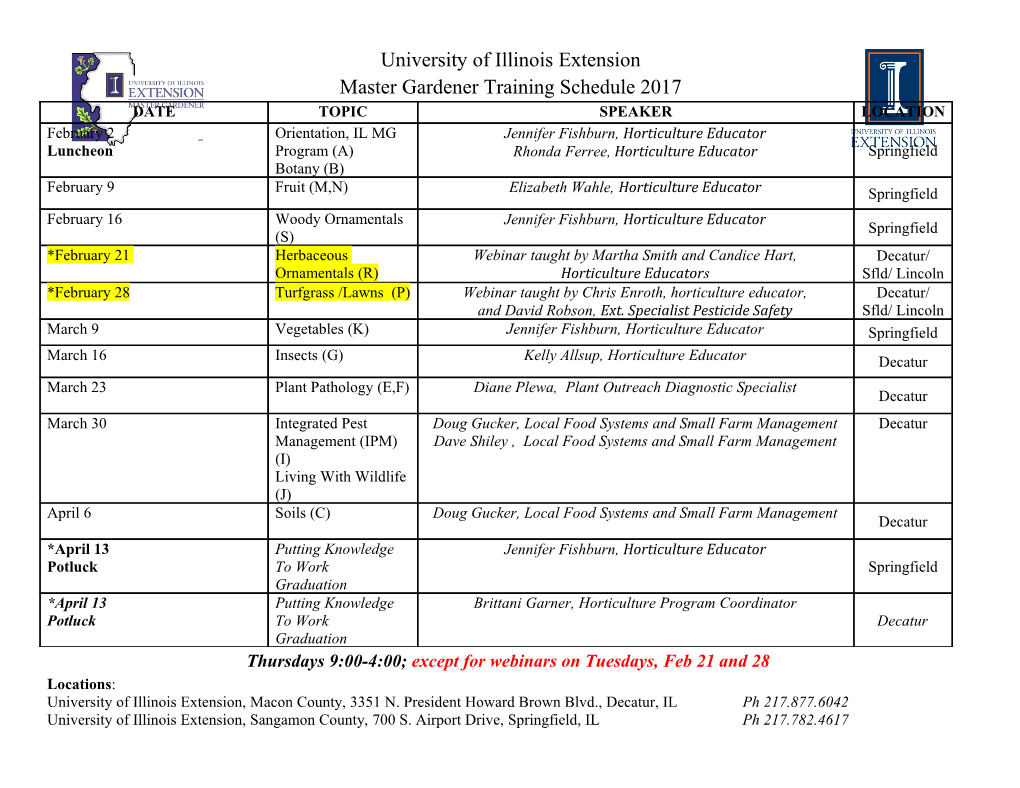
Theoretical and Experimental Investigation of Hall Thruster Miniaturization by Noah Zachary Warner S.B., Aeronautics and Astronautics, Massachusetts Institute of Technology, 2001 S.M., Aeronautics and Astronautics, Massachusetts Institute of Technology, 2003 SUBMITTED TO THE DEPARTMENT OF AERONAUTICS AND ASTRONAUTICS IN PARTIAL FULFILLMENT OF THE REQUIREMENTS FOR THE DEGREE OF DOCTOR OF PHILOSOPHY AT THE MASSACHUSETTS INSTITUTE OF TECHNOLOGY JUNE 2007 © 2007 Massachusetts Institute of Technology. All rights reserved. Signature of Author . Department of Aeronautics and Astronautics May 25, 2007 Certified by . Manuel Martínez-Sánchez Professor of Aeronautics and Astronautics Thesis Supervisor Certified by . Jack Kerrebrock Professor Emeritus of Aeronautics and Astronautics Certified by . Oleg Batishchev Principal Research Scientist in Aeronautics and Astronautics Certified by . Vladimir Hruby President, Busek Company, Inc. Accepted by . Jaime Peraire Professor of Aeronautics and Astronautics Chair, Committee on Graduate Students 2 Theoretical and Experimental Investigation of Hall Thruster Miniaturization by Noah Zachary Warner Submitted to the Department of Aeronautics and Astronautics on May 25, 2007 in partial fulfillment of the requirements for the degree of Doctor of Philosophy in Aeronautics and Astronautics in the field of Space Propulsion ABSTRACT Interest in small-scale space propulsion continues to grow with the increasing number of small satellite missions, particularly in the area of formation flight. Miniaturized Hall thrusters have been identified as a candidate for lightweight, high specific impulse propul- sion systems that can extend mission lifetime and payload capability. A set of scaling laws was developed that allows the dimensions and operating parameters of a miniaturized Hall thruster to be determined from an existing, technologically mature baseline design. The scaling analysis preserves the dominant plasma processes that deter- mine thruster performance including ionization, electron confinement and recombination losses. These scaling laws were applied to the design of a 9mm diameter, nominally 200W thruster based on the Russian D-55 anode layer Hall thruster. The Miniature Hall Thruster (MHT-9) design was further refined using magnetostatic and steady-state thermal finite element modeling techniques. Performance testing was conducted over a wide range of input powers from 20-500W with voltages between 100-300V and propellant flow rates of 0.3-1.0mg/s. Measured thrust was 1-18mN with a maximum thrust efficiency of 34% and specific impulse of 2000s. Significant erosion of thruster surfaces was observed due to the high plasma density required to maintain collisional mean free paths. Although the thrust efficiency was significantly lower than predicted by scaling laws, the MHT-9 is the best performing subcentimeter diameter Hall thruster built to date. A dimen- sionless performance analysis has shown that while the magnetic confinement ratio was successfully scaled, the thruster did not maintain the desired Knudsen number because of plasma heating. These trends were confirmed using a computational simulation. An ana- lytical model of electron temperature predicts that, due to a larger relative exposed wall area, the peak temperature inside the MHT-9 is higher than that of the D-55, resulting in greater ion losses and beam divergence. The inability to maintain geometric similarity was a result of the inherent challenges of maintaining magnetic field shape and strength at 3 4 ABSTRACT small scale, and this difficulty is identified as the fundamental limitation of Hall thruster miniaturization. Thesis Supervisor: Manuel Martínez-Sánchez Title: Professor of Aeronautics and Astronautics ACKNOWLEDGMENTS First and foremost, I would like to thank my research advisor and mentor, Professor Man- uel Martínez-Sánchez. When I entered MIT almost ten years ago as an undergraduate, the first class I enrolled in was a freshman seminar entitled "What’s in a Spacecraft?" Profes- sor Martínez-Sánchez was the instructor and has served as my advisor ever since. He has taught me how to be a scientist and shown me that a curious nature can lead to great dis- covery. I have truly appreciated his mentorship and friendship. I would like to thank my doctoral committee for their encouragement and support. Profes- sor Jack Kerrebrock brought the grounding and fundamental insight that is needed for every good research project. Dr. Oleg Batishchev’s knowledge of plasma physics and his warmhearted nature were very helpful. Dr. Vlad Hruby’s academic and financial contribu- tions were absolutely critical to the success of this project, and I will always be grateful for his support and encouragement. I would also like to thank my thesis readers, Professor Paulo Lozano and Dr. James Szabo. Paulo has helped me with various research projects since I was an undergraduate, and his contributions to this project were invaluable, particularly in the early design phase. He has also been a good friend for many years. James has taught me a great deal about how to successfully mix the worlds of theory and experiments, and I enjoyed the camaraderie we shared while working together at Busek. I would also like to thank Dr. Ray Sedwick who served as a surrogate advisor to me, offering both mentorship and financial support. There are several other folks at Busek who deserve my appreciation. Bruce Pote provided many insightful comments during the early design phase of the MHT-9 and has always been very supportive of my work. Larry Byrne was very helpful in setting up the experi- ments and served as a knowledgeable resource on how to successfully build and operate a low power Hall thruster. I would also like to thank Chaz Freeman and George Kolencik for their assistance with various aspects of the experimental work. I would like to acknowledge the support of the National Defense Science and Engineering Graduate Fellowship Program which is operated out of the Department of Defense. This program allowed me to pursue my own interests when otherwise not financially feasible. There are too many friends that I have made during my time at MIT to thank them all here. My fellow graduate students in both the Space Propulsion Laboratory and the Space Sys- tems Laboratory have been a wonderful resource both academically and socially. In partic- ular, I would like to thank Yassir Azziz and Shannon Cheng for their support and friendship over what often seemed like a very long road to the doctorate. We navigated every obstacle together as a team. I am not sure I could have made it this far without them, and I certainly would not have enjoyed it as much as I did. 5 6 ACKNOWLEDGMENTS My family has stood behind me every step of the way through my education and they have always been there to support me when needed. Every success of mine is simply a reflec- tion of their love and their belief in me and my abilities. I was incredibly fortunate to both meet and marry a beautiful woman during my time at MIT. My wife, Anjli, has always been a calming ocean of love and support, and I could not have done this without her. Not only did she never complain when I was spending late nights in the lab or the office, but she was always ready with a fresh perspective when I found myself in a difficult situation. I cannot thank her enough, but I offer her my sincere gratitude. NZW Cambridge May, 2007 TABLE OF CONTENTS Abstract . 3 Acknowledgments . 5 Table of Contents . 7 List of Figures . 11 List of Tables . 21 Nomenclature . 23 Chapter 1. Introduction . 27 1.1 Hall Thrusters . 28 1.1.1 Description . 28 1.1.2 Advantages . 31 1.1.3 Issues . 32 1.1.4 Wall Material and Geometry Variations . 33 1.2 Previous Work . 35 1.2.1 Low Power Hall Thrusters . 35 1.2.2 Scaling Theory . 38 1.3 Motivation . 40 1.4 Research Objectives . 41 1.5 Thesis Outline . 41 Chapter 2. Ideal Scaling of Hall Thrusters . 43 2.1 Ideal Scaling Theory . 44 2.1.1 Propellant Ionization . 45 2.1.2 Confinement of Electrons . 48 2.1.3 Power Lost to Discharge Chamber Walls . 54 2.1.4 Optimal Magnetic Field . 57 2.1.5 Thruster Power and Perimeter . 59 2.1.6 Cross Sectional Dimensions and Magnetic Field Strength . 60 2.1.7 Summary of Ideal Scaling Analysis . 61 2.1.8 Important Assumptions . 62 7 8 TABLE OF CONTENTS 2.2 Summary of Adopted Scaling Method . 64 2.3 Baseline Thruster Selection . 65 2.3.1 Baseline Thruster Candidates . 65 2.3.2 Considerations for Scaling . 65 2.3.3 Final Selection . 66 2.4 Scaling Results . 66 Chapter 3. Design of the MHT-9 . 69 3.1 Magnetic Circuit . 70 3.1.1 Field Requirements . 70 3.1.2 Components and Configuration . 71 3.1.3 Magnetic Material Selection . 78 3.1.4 Anode Tip Placement . 89 3.1.5 Magnetic Field Tuning . 91 3.2 Anode and Propellant Flow Design . 94 3.2.1 Flow Distribution . 94 3.2.2 Manufacturing . 96 3.2.3 Material Selection . 98 3.2.4 Insulation and Alignment . 99 3.3 Complete Design . 100 3.3.1 Propellant Connection and Isolation . 101 3.3.2 Discharge Power Connection . 106 3.3.3 Arcing . 107 3.4 Thermal Modeling . 110 3.4.1 Heat Load Estimates . 111 3.4.2 Thermal Contact Resistance . 120 3.4.3 Finite Element Model . 123 3.4.4 Model Results . 125 3.4.5 Design Improvements . 128 Chapter 4. Experimental Testing of the MHT-9 . 135 4.1 Experimental Setup . 135 4.1.1 Vacuum Tanks . 135 4.1.2 Thrust Stand . 137 4.1.3 Cathode . 138 4.1.4 Power . 139 4.1.5 Propellant . 140 4.1.6 Temperature Measurement . 141 TABLE OF CONTENTS 9 4.1.7 Data Collection . 142 4.2 Thruster Verification .
Details
-
File Typepdf
-
Upload Time-
-
Content LanguagesEnglish
-
Upload UserAnonymous/Not logged-in
-
File Pages264 Page
-
File Size-