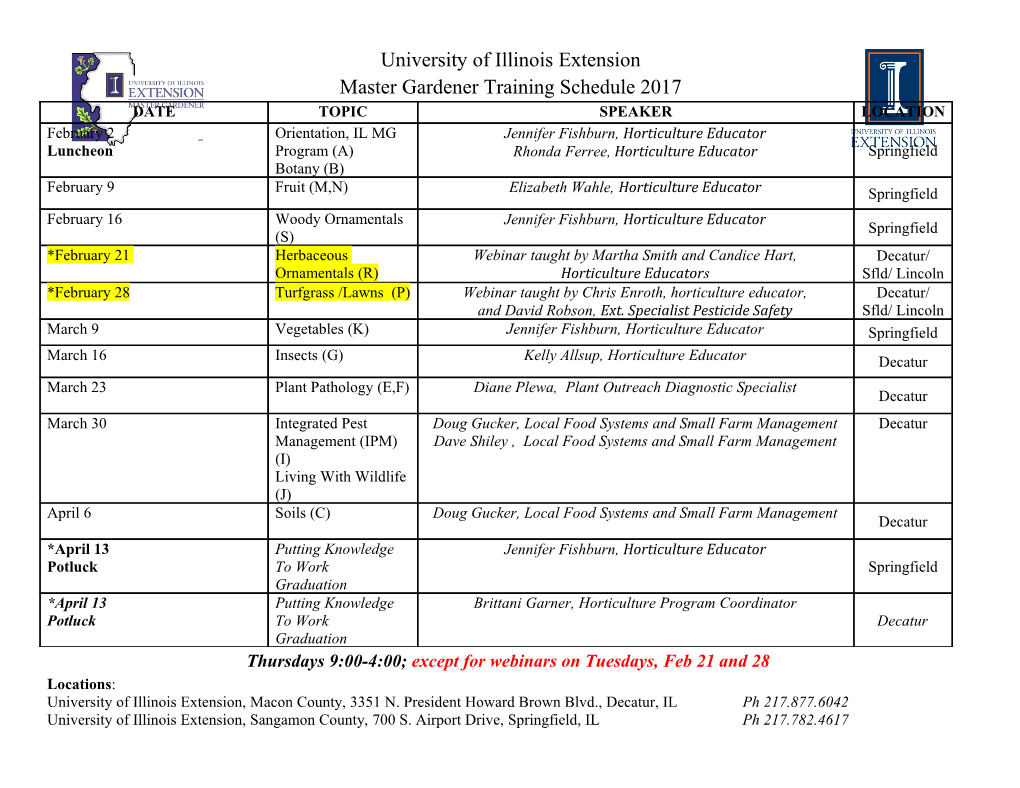
This article appeared in a journal published by Elsevier. The attached copy is furnished to the author for internal non-commercial research and education use, including for instruction at the authors institution and sharing with colleagues. Other uses, including reproduction and distribution, or selling or licensing copies, or posting to personal, institutional or third party websites are prohibited. In most cases authors are permitted to post their version of the article (e.g. in Word or Tex form) to their personal website or institutional repository. Authors requiring further information regarding Elsevier’s archiving and manuscript policies are encouraged to visit: http://www.elsevier.com/copyright Author's personal copy Physics of the Earth and Planetary Interiors 187 (2011) 225–231 Contents lists available at ScienceDirect Physics of the Earth and Planetary Interiors journal homepage: www.elsevier.com/locate/pepi Evolving core conditions ca. 2 billion years ago detected by paleosecular variation ⇑ Aleksey V. Smirnov a, , John A. Tarduno b,c, David A.D. Evans d a Department of Geological and Mining Engineering and Sciences, Michigan Technological University, Houghton, MI 49931, USA b Department of Earth and Environmental Sciences, University of Rochester, Rochester, NY 14627, USA c Department of Physics and Astronomy, University of Rochester, Rochester, NY 14627, USA d Department of Geology and Geophysics, Yale University, New Haven, CT 06520, USA article info abstract Article history: Paleomagnetic data provide one of the few probes available to interrogate early evolution of the core. Received 13 January 2011 Here we apply this probe by examining the latitudinal dependence of paleosecular variation (PSV) data Received in revised form 9 May 2011 derived from high-quality paleomagnetic data collected from Proterozoic and Neoarchean rocks. These Accepted 15 May 2011 data define a Neoarchean geomagnetic field that was more dipolar than that during Proterozoic times, Available online 30 May 2011 indicating a change in core conditions. The signals observed may reflect a change in forcing of the Guest Editor: Pan Yongxin. Edited by: Keke Zhang. dynamo and an early onset of inner core growth. We propose a model that links evolution of the core, mantle and crust in three principal phases: (i) Before approximately 3.5 Ga, an entirely liquid core may not have hosted a geodynamo. If heat transport was sufficient across the core–mantle boundary, Keywords: Geodynamo however, a geodynamo could have been generated. If so, sources in the shallow outer core could have Secular variation been more important for generating the dynamo relative to deeper convection, resulting in a field that Inner core was less dipolar than that generated in later times. (ii) Cooling of the lower mantle between ca. 2 and 3.5 billion years ago was promoted by deep subduction and possibly coincided with inner core growth. The geodynamo during this episode was deeply-seated producing a highly dipolar surface magnetic field. (iii) After ca. 2 billion years ago, continued subduction led to large-scale core–mantle boundary compo- sitional and heat flux heterogeneity. With these changes, shallow core contributions to the geomagnetic field grew in importance, resulting in a less dipolar field. Ó 2011 Elsevier B.V. All rights reserved. 1. Introduction where N is the number of VGPs and Di is the angle between the ith VGP and the mean paleomagnetic pole. McFadden et al. (1991) There is currently much debate over the nature of Earth’s early modeled S as independent dipole (SD, antisymmetric) and quadru- core. For example, estimates for the onset of solid inner core nucle- pole (SQ, symmetric) families, with the latter dominating at the ation range from times younger than 1 Ga (Aubert et al., 2009)to equator: 3.5 Ga (Gubbins et al., 2004). Paleointensity data indicate the pres- qffiffiffiffiffiffiffiffiffiffiffiffiffiffiffiffi qffiffiffiffiffiffiffiffiffiffiffiffiffiffiffiffiffiffiffiffiffiffi ence of a geodynamo in Mesoarchean and Paleoarchean times 2 2 2 2 SðkÞ¼ SD þ SQ ¼ ðakÞ þ b ð2Þ (3.2–3.45 Ga) (Tarduno et al., 2007, 2010). But some models sug- gest that strong fields can be generated by an early dynamo with- where k is paleolatitude, and a and b are constants. Complete inde- out inner core growth (Sakuraba and Kono, 1999). We can gain pendence of the two families is unlikely, but this interpretation insight into early core conditions by examining the morphology (Model G) remains a useful framework to gauge past paleosecular of the ancient geomagnetic field defined by paleomagnetic data. variation (PSV). Specifically, we can track the importance of non-dipole fields in Although lava flow sequences have yielded high resolution PSV the past using the angular dispersion (S) of virtual geomagnetic values for the last 5 million years (e.g., Johnson et al., 2008), data poles (VGPs) derived from paleomagnetic data: on billion-year time scales are more difficult to obtain. A few ex- vffiffiffiffiffiffiffiffiffiffiffiffiffiffiffiffiffiffiffiffiffiffiffiffiffiffiffi tant lava flow sequences are available, but these must be supple- u u XN mented with data from dike swarms. Any given regional data set t 1 2 S ¼ D ð1Þ may fortuitously overestimate or underestimate PSV. But if data N À 1 i i¼1 sets are available spanning many latitudinal belts from multiple ancient cratons, a synoptic view of PSV can be derived. Smirnov and Tarduno (2004) found that such data suggest that the field at ⇑ Corresponding author. Tel.: +1 906 487 2365. the time of the Proterozoic/Archean boundary (2.5 Ga) was more E-mail address: [email protected] (A.V. Smirnov). dipolar than the field of the last 5 million years. This result was 0031-9201/$ - see front matter Ó 2011 Elsevier B.V. All rights reserved. doi:10.1016/j.pepi.2011.05.003 Author's personal copy 226 A.V. Smirnov et al. / Physics of the Earth and Planetary Interiors 187 (2011) 225–231 confirmed by an analysis of the same time window by Biggin et al. P3 samples. (2) Data must be from modern demagnetization (2008a). Here, we expand our initial analysis, to assess any PSV and processing techniques (e.g., principal component analysis). pattern that might reflect changes in the Precambrian core (3) A primary origin of the magnetization must be convincingly conditions. demonstrated. (4) Data must be consistent with a thermorema- nent magnetization, without evidence of chemical remanence. (5) Magnetization age must be reliably constrained. No site selec- 2. Application and results tion criteria based on the precision parameter (k) or maximum 95% confidence area (a95) were applied. However, for most (575 We have identified two Precambrian time windows where glo- of 585) of the accepted sites, a95 did not exceed 20°. bal igneous units allow a new assessment (Table 1; Fig. 1A). We S values were corrected for within-site dispersion following used the Global Paleomagnetic Database GPMDB4-6 (Pisarevsky, Doell (1970). In three studies, published information is insufficient 2005)(www.tsrc.uwa.edu.au/data_bases), supplemented with to correct S, but the large number of samples and site-level statis- recent results for our new data set. We exclude data from sedimen- tics lead us to believe that any additional uncertainty is less than a tary, metamorphic, plutonic and silicic extrusive rocks. In particu- few degrees. S confidence intervals (1r) were calculated using a lar, the silicic lavas often do not form easily distinguishable lava N À 1 jackknife method (Efron, 1982). flows and may be deposited on slopes, which makes it difficult to As opposed to the small, select Matachewan dike data set used assess the number of independent cooling units and structural by Smirnov and Tarduno (2004), we use a new compilation (Evans corrections. Therefore, our analysis was confined to mafic and and Halls, 2010); directions from the western subprovince of the intermediate extrusive rocks and shallow mafic intrusions that Superior craton were rotated using an Euler pole at 51°N, 85°W can record distinct field directions. We further apply the following and rotation angle of 14°CW. We exclude results from the Derde- criteria: (1) Directions must be from P10 sites each comprising poort basalts (Wingate, 1998) used by Biggin et al. (2008a) because Table 1 Summary of paleomagnetic studies used for estimating the paleosecular variation. Unit Age (Ma) B Plat S ±dS C/UC Sexp Sobs À Sexp Sign Bangemall Basin Sillsa 1070 11 27.8 13.9 ± 2.6 C 11.6 2.3 + Lake Shore trapsb 1087 30 14.6 13.6 ± 0.8 C 10.6 3.0 + Portage Lake Volcanicsc 1095 28 16.9 14.5 ± 1.2 UC 10.7 3.8 + North Shore Trapsd 1098 34 27.3 11.4 ± 2.1 C 11.5 À0.1 À Mamainse Point Upper Ne 1100 21 22.4 4.8 ± 3.0 C 11.1 À6.3 À Umkondo doleritesf 1110 15 6.8 14.2 ± 3.1 C 10.3 3.9 + Cleaver dikesg 1740 17 39.1 14.4 ± 4.0 C 12.8 1.6 + Taihang dikesh 1769 19 2.6 8.5 ± 2.6 C 10.2 À1.7 À Flaherty volcanicsi 1870 11 26.2 11.6 ± 1.7 UC 11.4 0.2 + Mashonaland doleritesj 1880 16 28.8 14.8 ± 4.0 C 11.7 3.1 + Fort Frances dikesk,l 2067–2077 12 35.1 11.2 ± 3.3 C 12.3 À1.1 À Marathon dikes (R)k,l 2101–2106 13 37.0 14.0 ± 2.5 C 12.6 1.4 + Marathon dikes (N)k,l 2121–2126 16 39.9 16.0 ± 2.6 C 12.9 3.1 + Biscotasing dikesm 2169 12 38.4 12.6 ± 2.3 C 12.7 À0.1 À Ongeluk lavasn 2200 32 14.1 7.1 ± 7.5 C 10.5 À3.4 À Dharwar dikeso 2367 25 69.1 15.5 ± 1.1 C 17.1 À1.6 À Matachewan dikes (N)p 2473–2446 28 14.8 6.4 ± 2.9 C 10.6 À4.2 À Matachewan dikes (R)p 2473–2446 101 7.7 8.8 ± 5.1 C 10.3 À1.5 À Karelia dikesq 2440 11 30.0 10.7 ± 1.3 UC 11.8 À1.1 À Allanridge lavasr,s 2664–2709 17 43.2 12.1 ± 5.7 C 13.3 À1.2 À Upper Fortescue lavast 2715 16 35.0 12.9 ± 3.2 C 12.3 0.6 + Fortescue Lower lavast 2746 75 49.5 12.8 ± 2.0 C 14.2 À1.4 À Fortescue Package 0t >2772 24 58.3 16.1 ± 2.8 C 15.4 0.7 + B: number of units; Plat: paleolatitude; S, dS: angular dispersion of VGPs and confidence interval; C/UC: data corrected (uncorrected) for within-site dispersion; Sobs À Sexp: difference between observed S and that predicted (Sexp) from Model G fit to all data (a = 0.20 ± 0.04, b = 10.17 ± 0.90); Sign: result of Sign Test (see text).
Details
-
File Typepdf
-
Upload Time-
-
Content LanguagesEnglish
-
Upload UserAnonymous/Not logged-in
-
File Pages8 Page
-
File Size-