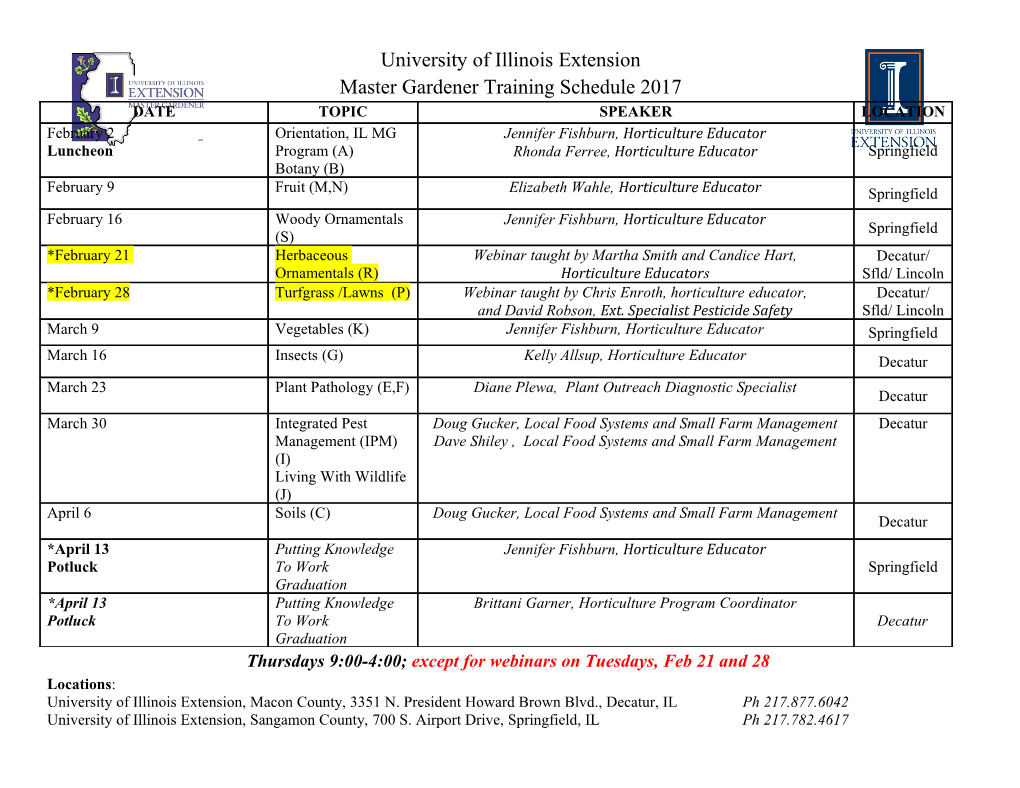
Edinburgh Research Explorer Post-depositional fracturing and subsidence of pumice flow deposits: Lascar Volcano, Chile Citation for published version: Whelley, PL, Jay, J, Calder, ES, Pritchard, ME, Cassidy, NJ, Alcaraz, S & Pavez, A 2012, 'Post-depositional fracturing and subsidence of pumice flow deposits: Lascar Volcano, Chile' Bulletin of Volcanology, vol 74, no. 2, pp. 511-531. DOI: 10.1007/s00445-011-0545-1 Digital Object Identifier (DOI): 10.1007/s00445-011-0545-1 Link: Link to publication record in Edinburgh Research Explorer Document Version: Publisher's PDF, also known as Version of record Published In: Bulletin of Volcanology Publisher Rights Statement: This is an Open-Access article distributed under the terms of the Creative Commons Attribution License, which permits unrestricted use, distribution, and reproduction in any medium, provided the original author and source are properly cited. General rights Copyright for the publications made accessible via the Edinburgh Research Explorer is retained by the author(s) and / or other copyright owners and it is a condition of accessing these publications that users recognise and abide by the legal requirements associated with these rights. Take down policy The University of Edinburgh has made every reasonable effort to ensure that Edinburgh Research Explorer content complies with UK legislation. If you believe that the public display of this file breaches copyright please contact [email protected] providing details, and we will remove access to the work immediately and investigate your claim. Download date: 28. Apr. 2017 Bull Volcanol (2012) 74:511–531 DOI 10.1007/s00445-011-0545-1 RESEARCH ARTICLE Post-depositional fracturing and subsidence of pumice flow deposits: Lascar Volcano, Chile Patrick L. Whelley & J. Jay & E. S. Calder & M. E. Pritchard & N. J. Cassidy & S. Alcaraz & A. Pavez Received: 11 September 2010 /Accepted: 22 August 2011 /Published online: 27 September 2011 # Springer-Verlag 2011 Abstract Unconsolidated pyroclastic flow deposits of the radar interferometry shows that deposit subsidence of up to 1993 eruption of Lascar Volcano, Chile, have, with time, 1 cm/year occurred between 1993 and 1996 with continued become increasingly dissected by a network of deeply subsidence occurring at a slower rate thereafter. In situ penetrating fractures. The fracture network comprises measurements show that 1 m below the surface, the 1993 orthogonal sets of decimeter-wide linear voids that form a deposits remain 5°C to 15°C hotter, 18 years after pseudo-polygonal grid visible on the deposit surface. In this emplacement, than adjacent deposits. Based on the observed work, we combine shallow surface geophysical imaging subsidence as well as estimated cooling rates, the fractures are tools with remote sensing observations and direct field inferred to be the combined result of deaeration, thermal measurements of the deposit to investigate these fractures contraction, and sedimentary compaction in the months to and their underlying causal mechanisms. Based on ground years following deposition. Significant environmental factors, penetrating radar images, the fractures are observed to have including regional earthquakes in 1995 and 2007, accelerated propagated to depths of up to 10 m. In addition, orbiting settling at punctuated moments in time. The spatially variable fracture pattern relates to surface slope and lithofacies Editorial responsibility: B. van Wyk de Vries variations as well as substrate lithology. Similar fractures Electronic supplementary material The online version of this article have been reported in other ignimbrites but are generally (doi:10.1007/s00445-011-0545-1) contains supplementary material, exposed only in cross section and are often attributed to which is available to authorized users. formation by external forces. Here we suggest that such P. L. Whelley (*) : E. S. Calder interpretations should be invoked with caution, and deforma- Department of Geology, University at Buffalo, tion including post-emplacement subsidence and fracturing of State University of New York, loosely packed ash-rich deposits in the months to years post- 411 Cooke Hall, emplacement is a process inherent in the settling of pyroclastic Buffalo, NY 14260-3050, USA e-mail: [email protected] material. : J. Jay M. E. Pritchard Keywords Lascar . Ignimbrite . InSAR . Ground penetrating Department of Earth as Atmospheric Sciences, Cornell University, radar . Compaction . Deaeration . Cooling Ithaca, NY, USA N. J. Cassidy School of Physical and Geographical Sciences, Keele University, Introduction Keele, UK S. Alcaraz We document a network of fractures, which are progres- Department of Earth Sciences, The Open University, sively dissecting the 18–20 April 1993 pumice flow Milton Keynes MK7 6AA, UK deposits at Lascar Volcano, Chile (Fig. 1). The fractures are manifest at the surface as elongate irregular trenches, A. Pavez Departamento de Geofísica, Universidad de Chile, which cut through unconsolidated ignimbrite. They repre- Santiago, Chile sent the first stages of post-depositional deformation of 512 Bull Volcanol (2012) 74:511–531 Fig. 1 a The location of Lascar Volcano in northern Chile. b a b Active vent View of Lascar from an over- Lascar looking cliff to the north showing the northwest fan of 1993 deposits. c Individual pyroclastic flow units on break- out fans I and II, mapped on a portion of GEOTEC image 14602-04. Relative stratigraphic positions were deduced by field observations Breakout fan I Breakout fan II 200 m c 13 4 14 Fan I 6 3 13 14 5 11 12 2 Lava Flow 11 10 1 5 12 1 7 9 9 3 8 Relative age 7 8 2 6 Fan II 10 5 6 6 5 S 4 2 3 4 4 3 1 2 Relative age 1 100 m ignimbrite in an arid environment. Their presence at Lascar continuum must exist between deaeration and the longer- provides an opportunity to map their distribution, monitor term effects of compaction involving reduction of void space their development, and constrain the timescales over which and grain reorientation, but this has never been specifically their formative processes operate. This work furthers the investigated. Field observations of easily fluidized fresh understanding of how pyroclastic materials behave and are deposits are common (Rowley et al. 1981; Wilson and Head modified by, or respond to, environmental factors in the 1981; Torres et al. 1996; Branney and Kokelaar 2002;P. decades after emplacement. Cole, personal communication, 1997) and indicate that at the Post-depositional deformation of pyroclastic deposits natural scale, deaeration must continue over longer time occurs over a range of spatial and temporal scales (Table 1). periods (days to weeks) than can be reproduced in the Internally driven processes, such as deaeration, cooling, laboratory (e.g., Druitt et al. 2004, 2007). initial compaction, and the onset of welding, are dependent External forces drive additional processes which may on the eruptive conditions and material properties, including affect those already mentioned: Seismic ground accelera- grain size and composition. These processes begin to occur tion can induce fracturing (e.g., Nocita 1988; Brown and during and immediately after emplacement, but the timescales Branney 2004), circulating fluids or volatiles can cement or over which they extend are not well constrained. The deposits indurate unconsolidated deposits (e.g., Zies 1924; Bacon studied here are non-welded and largely unconsolidated, so 2008; Calder et al. 2000), and mass wasting and fluvial or welding, which is extensively covered elsewhere (e.g., Ross eolian erosion modify the aspect of the deposits. Mass and Smith 1961; Wolff and Wright 1981;Sparksetal.1999), wasting and fluvial erosion can also induce secondary flow is not considered here. Recent laboratory experiments on phenomena including deposit-derived pyroclastic flows fluidized natural samples (e.g., Druitt et al. 2004, 2007) (Torres et al. 1996), debris flows, and hyper-concentrated found that deaeration reduced deposit thickness by 50%, in lahars (e.g., Pierson et al. 1992; Branney and Gilbert 1995; the minutes after emplacement. In natural systems, a Major et al. 1996). The deposit-derived pyroclastic flows at Bull Volcanol (2012) 74:511–531 513 Table 1 Post-depositional processes affecting ignimbrites Process Time scale Features/effects Example Reference Internal Deaeration Minutes to years Density increase Mt. St. Helens, Wilson and Head 1981; (lithological) laboratory experiments Rowley et al. 1981;Druittetal. 2004, 2007 Welding Minutes to days Eutaxitic fabric, Bishop Tuff, Murga Ross and Smith 1961; fiamme, joints Caldera Ignimbrites Sparks et al. 1999 Rheomorphism Minutes to hours Flow lineations, deposit Borrowdale Volcanic Schmincke and Swanson to days thickness variations, Group, TL Ignimbrite 1967; Sumner and eutaxitic fabric Branney 2002; Branney et al. 2004 Compaction Weeks to decades Fracture network, Grants Ridge Tuff, Keating and Valentine subsidence Lascar 1998; this work pumice flow deposit Cooling Years Joints Bishop Tuff Sheridan 1970 Indurationa Decades to millions Hardening, fumarolic Valley of 10,000 Smokes; Griggs 1922; Sheridan of years incrustations, mounds Bishop Tuff; Akdag- 1970; Schumacher and and ridges Zelve Ignimbrite; Mues-Schumacher 1997; Mazama-Crater Bacon 2008 Lake External Aeolian erosion Days to millions Yardangs, pits Tara Ignimbrite Bailey et al. 2007 (environmental) of years Fluvial erosion Days to decades Gullies, channels Atana Ignimbrite Bailey et al. 2007 Mass wasting Days to millennia Fractures,
Details
-
File Typepdf
-
Upload Time-
-
Content LanguagesEnglish
-
Upload UserAnonymous/Not logged-in
-
File Pages22 Page
-
File Size-