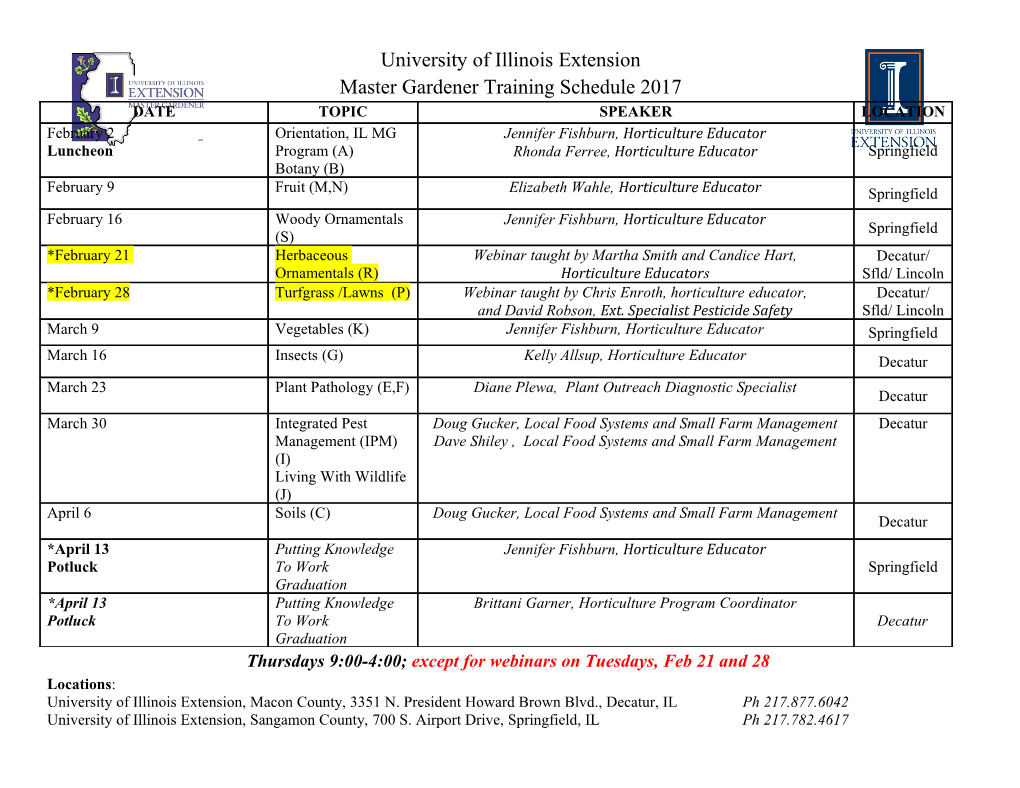
CALIFORNIA STATE UNIVERSITY, NORTHRIDGE EVOLUTION OF ANTHOCYANIDIN SYNTHASE IN HAWAIIAN SILVERSWORDS AND CALIFORNIA TARWEEDS A thesis submitted in partial fulfillment of the requirements for the degree of Master of Science in Biology by Ekaterina Kovacheva May 2011 The thesis of Ekaterina Kovacheva is approved: Michael Summers, Ph.D. Date Stan Metzenberg, Ph.D. Date Virginia berholzer Vandergon, Ph.D., Chau Date California State University, Northridge 11 TABLE OF CONTENTS Signature Page 11 List ofFigures IV Abstract v Introduction 1 Materials and Methods 9 Results 15 Discussion 19 Conclusion 24 References 25 Appendix A: Figures 31 Appendix B: Coding Sequences and Multiple Sequence Alignments 49 111 LIST OF FIGURES Figure Al: A generalized scheme ofthe flavonoid biosynthetic pathway 31 Figure A2: Proposed mechanism of action of anthocyanidin synthase 32 Figure A3. Comparison of the action of iron and 2-0G dependent oxygenases ANS and FLS 3 3 Figure A4: Structure of ANS 34 Figure AS: Map of ANS and primers used 35 Figure A6: Primers that successfully produced results 36 Figure A7: Species used for pylogenetic analysis 37 Figure A8: PCR Protocol for all reactions 39 Figure A9: Cycling conditions for PCR 40 Figure AlO: Number of forward and reverse clones 41 Figure All: Intron sizes and sections of exons 1 and 2 that were sequenced from each copy of ANS 42 Figure Al2: Maximum likelihood tree generated with MEGA 5 43 Figure A13: Bayesian tree generated with MrBayes 3.1.2 45 Figure Al4: Ka/Ks values for selected Madiinae 47 Figure Al5: Ka/Ks ratios for various structural and regulatory genes found in Madiinae 48 Figure B 1: Coding sequences obtained in this study 49 Figure B2: Protein sequence aligmnent of all sequences used in this study 52 Figure B3: Protein sequence alignment of the sequences, excluding the short Holocarpha sp. 54 Figure B4: DNA alignment of all sequences used in this study 56 Figure B5: DNA aligmnent of the longest sequences used in this study 60 lV ABSTRACT EVOLUTION OF ANTHOCYANIDIN SYNTHASE IN HAWAIIAN SILVERSWORDS AND CALIFORNIA TARWEEDS By Ekaterina Kovacheva Master of Science in Biology One of the most spectacular examples of insular adaptive radiation can be found among the Hawaiian silverswords (Asteraceae-Madiinae). This monophyletic group was transported to the Hawaiian archipelago about five million years ago, and today comprises 32 species in three genera. Having to adapt to very varied and dynamic environments, the plants display stunning morphological and physiological diversity. However, their genotypic diversity is low, as evidenced by the ease with which species of different genera form hybrids in nature and in the lab. This discrepancy can be explained by differences in just a few key loci, which could modify entire pathways. The closest relatives of the silverswords are thought to be the tarweeds (Asteraceae-Madiinae) on the west coast ofNorth America. Two tarweed ancestors hybridized to form the allotetraploid ancestor of all known silverswords, which was then transported to the Hawaiian islands, most likely by a bird. The pathway I am concemed with is the anthocyanin-producing pathway, in particular anthocyanidin synthase (ANS), a late gene in the pathway. Anthocyanins are pigments responsible for blue, red and purple color in flowers. However, due to their many other functions, such as light attenuation in chloroplasts, I expect the genes of this pathway, including ANS, to be intact in most silverswords, even though only one species has red flowers. I also expect to find two copies in silverswords due to tetraploidy. Degenerate primers were designed in conserved regions of ANS and PCR was done to obtain the gene from two tarweeds (Madia elegans, Madia gracilis) and two silverswords (Dubautia linearis, Wilkesia gymnoxiphium). Gene sequences were spliced and edited with BioEdit, and were aligned with ClustalW 1.8. A maximum likelihood tree was made with MEGA 5, and a bayesian tree was made with MrBayes 3 .1.2. A Z-test of selection and a Ka/Ks test were done using MEGA 5. A RACE reaction wasperfonned on mRNA from the flowers of M elegans. The entire gene (except 20bp of primers in the flanking regions) of two exons and one intron was sequenced from all species studied. The RACE in M elegans produced the entire gene, and showed there was some expression in the floral tissues of this plant. As expected, two copies were found in the silverswords and only one in each of the tarweeds. No premature stop codons were found within coding sequences. Phylogenetic v analysis groups the Madiinae separately from other Asteraceae, and the tarweeds separately from the silverswords. Within the siverswords, copy 1 from both species is separate from copy 2 from both species. Interestingly, intron size seems to be conserved within the tarweeds and copy 2 (547bp), and also within copy 1 (485bp). Purifying selection was detected in the silversword copies, while no positive selection was found in any copy. The positions of all copies studied and their relative position to other Asteraceae support the allopolyploid origin of the silverswords. Each copy in the silverswords most likely came from each of the two mainland ancestors. Given the overall similarity of the sequences within all Madiinae, it is reasonable to conclude that the gene starts and ends in the same position in all Madiinae. The lack of premature stop codons indicates that the gene may be at the very least functional, although we have no knowledge of its level of expression within the floral or other tissues. The flower color of all plants in this study is yellow, indicating no accumulation of anthocyanins, although they may be found in other tissues. The lab is cunently investigating the sequences of other genes in the pathway, and future studies will focus on sequencing the promoter region and known regulators of ANS expression. This will give us a more complete picture of the evolution of the pathway, helping us understand the genetic mechanisms behind adaptive radiations. Vl INTRODUCTION The anthocyanin biosynthetic pathway The anthocyanin biosynthetic pathway (ABP) is most well-known for its role in coloration and other important functions of plants, as well as for plants' health benefits to humans, and as such it has been very well-studied (Grotewold, 2006; Koes, Quattrocchio, & Mol, 1994; Winkel-Shirley, 2001). The earliest known research focused on anthocyanins (ANs) as pigments, and included studies ofthe effects of acids and bases on pigment colors (Winkel, 2006; Winkel-Shirley, 2001) and on the inheritance pattems of flower and seed color (MendelWeb, 1997). In the 1910s it was found that pigments in many plants are derived from three basic anthocyanidins: pelargonidin, cyanidin and delphinidin (Winkel, 2006). At that time it was also established that these compounds were chemically related to the flavonols quercetin, kaempferol and myricetin, and that they sometimes contained sugar or methoxy groups (Winkel, 2006; Winkel-Shirley, 2001 ). All of these compounds belong to the group of chemicals known as flavonoids; ABP is part of the larger flavonoid biosynthetic pathway. The 1950s and 1960s saw an increase in the understanding of flavonoid structures and in the genetic and enzymatic data of genes involved in their biosynthesis (Winkel, 2006). By the 1990s, the major enzymes in the pathway, including anthocyanidin synthase, the focus of this study, had been indentified and their structures solved (Saito, et al., 1999). An overview of the generalized flavonoid biosynthetic pathway is shown in Figure Al. Chalcone synthase (CHS) catalyzes the first committed step by condensing three molecules ofMalonyl-CoA with a molecule ofp-Coumaroyl-CoA to produce tetrahydroxychalcone (Grotewold, 2006; T. A. Holton & Comish, 1995). Chalcone isomerase (CHI) then isomerizes the chalcone by closing the C-ring, producing the flavanone naringenin. Flavanone 3-hydroxylase (F3H) converts naringenin to dihydrokaempferol (DHK), which is then converted to leucopelargonidin (a leucoanthocyanidin) by dihydroflavonol reductase (DFR). Leucoanthocyanidins are converted to colored anthocyanidins by anthocyanidin synthase (ANS, synonym: leucoanthocyanidin dioxygenase, LDOX). The resulting anthocyanidins are unstable; glycosylation at C-3 and/or C-5 converts them to anthocyanins and stabilizes them. Finally, glutathione S-transferase (GST) mediates the transport of ANs to the vacuole. As seen in Figure A1, there is multiple branching of the pathway. DHK can be hydroxylated by flavonoid 3 '-hydroxylase (F3 'H) to make dihydroquercetin (DHQ), and both DHK and DHQ can be converted to dihydromyricetin (DHM) by flavonoid 3 '5 '­ hydroxylase (F3 '5 'H). Subsequently, DHQ leads to cyanidin, and DHM to delphinidin. DFR is known to have some substrate specificity (Helariutta, et al., 1993; Katsumoto et al., 2007; Meyer, et al., 1987), but no such specificity has been observed in ANS. The resulting anthocyanidins differ by the number of hydroxyl groups on the B-ring and by their color; pelargonidins are typically brick red, cyanidins are purple, and delphinidins are blue. Some of the precursors of anthocyanidins lead to other important flavonoid compounds. All of the substrates ofDFR can be utilized by flavonol synthase (FLS) to produce flavonols, and flavone synthase (FNS) can produce flavones from naringenin. 1 These flavonoids have a myriad of other functions in plants, but more importantly they can contribute to color stability by serving as co-pigments for anthocyanins (Grotewold, 2006). Evolution ofFlavonoids Flavonoids most likely appeared in stages as plants migrated to land (Koes, et al., 1994; Stafford, 1991). Chalcones, flavanones and flavonols first appeared in the ancestors of modem Bryophytes, about 500 million years ago (mya), although not all modem Bryophytes contain flavonoids. These compounds are today found across the entire plant kingdom (Stafford, 1991). Proanthocyanidins appeared in ferns 370mya (Koes, et al., 1994; Stafford, 1991). Anthocyanins did not appear until120mya, and are today found in some gymnosperms and in all angiosperms (Stafford, 1991), except the Caryophyllales (Stafford, 1994).
Details
-
File Typepdf
-
Upload Time-
-
Content LanguagesEnglish
-
Upload UserAnonymous/Not logged-in
-
File Pages68 Page
-
File Size-