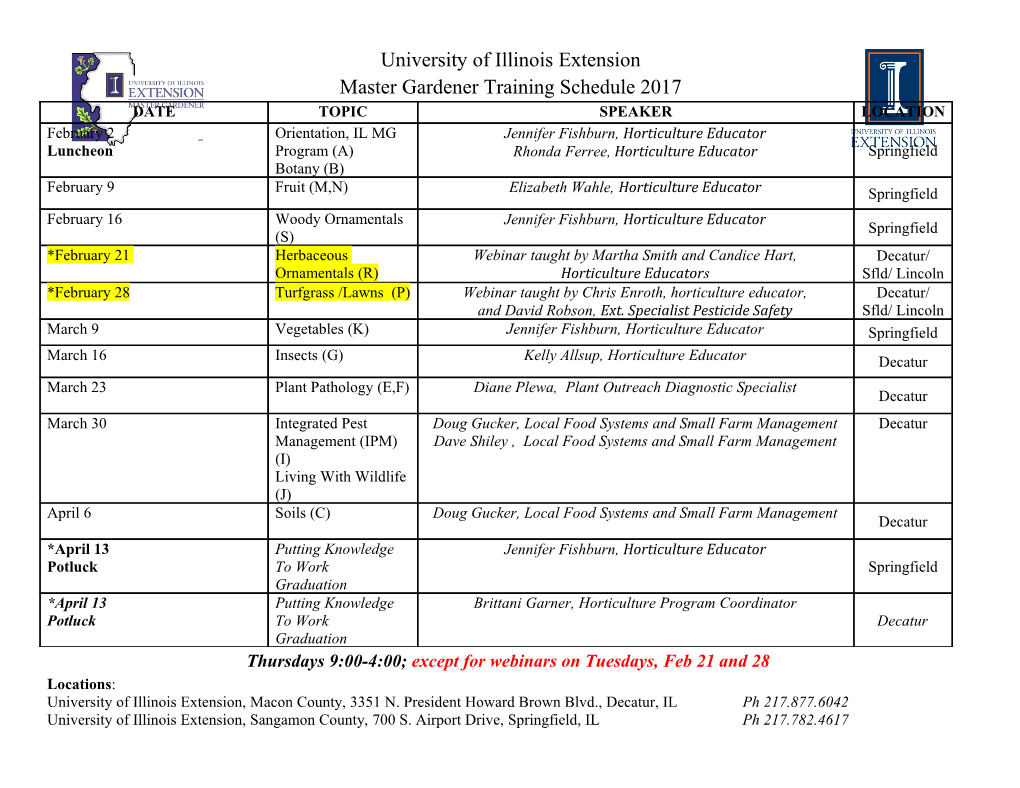
This is an electronic reprint of the original article. This reprint may differ from the original in pagination and typographic detail. Skrobo, Benedikt; Rolfes, Julian D.; Deska, Jan Enzymatic approaches for the preparation of optically active non-centrochiral compounds Published in: Tetrahedron DOI: 10.1016/j.tet.2016.01.026 Published: 10/03/2016 Document Version Peer reviewed version Please cite the original version: Skrobo, B., Rolfes, J. D., & Deska, J. (2016). Enzymatic approaches for the preparation of optically active non- centrochiral compounds. Tetrahedron, 72(10), 1257-1275. https://doi.org/10.1016/j.tet.2016.01.026 This material is protected by copyright and other intellectual property rights, and duplication or sale of all or part of any of the repository collections is not permitted, except that material may be duplicated by you for your research use or educational purposes in electronic or print form. You must obtain permission for any other use. Electronic or print copies may not be offered, whether for sale or otherwise to anyone who is not an authorised user. Powered by TCPDF (www.tcpdf.org) 1 Enzymatic Approaches for the Preparation of Optically Active Non-Centrochiral Compounds Benedikt Skrobo, Julian D. Rolfes and Jan Deska* Department of Chemistry, Aalto-yliopisto, FI-02150 Espoo, Finland ARTICLE INFO Article history: Received Received in revised form Accepted Available online Keywords: Biocatalysis Axial Chirality Planar Chirality Helicity Stereochemistry Contents 1. Introduction ………………………………………………………………… 2. Lipases and esterases as catalysts …………………………………………………..... 2.1 Axial chirality 2.2 Helical chirality ……………………………………………………………………… 2.3 Planar chirality ……………………………………………………………………… 3. Oxidoreductases as catalysts ………………………………………………………… 3.1 Axial chirality ……………………………………………………………………… 3.2 Planar chirality ……………………………………………………………………… 4. Miscellaneous biocatalytic systems ………………………………………………… 5. Conclusion ………………………………………………………………………......... 1. Introduction atropisomerism is for example met in certain biaryls,1 overcrowded olefins,2 anilides3 and other helically twisted Central chirality is ubiquitously found in molecular molecules. Moreover, non-central stereogenicity is observed architectures and the basics of asymmetrically tetrasubstituted when a suitable set of substituents at formally planar structural carbon atoms are a vital part in every first year organic chemistry subunits opens up a spatial arrangement to allow for molecules to undergraduate course. However, in addition to that a variety of exist as image and mirror image. This broader term of static other stereochemical elements are widespread both in the inherent chirality covers axially chiral allenes4 and macrosopic world and on the molecular level. Despite the lack of alkylidenecycloalkanes as well as planar chiral cyclophanes,5 appropriately decorated tetrahedral units, numerous structural metallocenes,6 and calixarenes,7 among others. features can lead to non-superimposable sets of enantiomeric molecules. On one side, inherent chirality can occur when free Although not always self-evident at first glance and rotation is hindered due to steric or electronic constraints and the sometimes accompanied by a touch of chemical esotericism, all energy barrier for the interconversion of individual conformers these aspects of chirality are not just artificial constructs but can prohibits rapid epimerization. The requirement for such an be rather widely found in Nature, in the screw sense of biological macromolecules and likewise on the small-molecule level. * Corresponding author. E-mail address: [email protected] (J. Deska) Hence, a great number of structurally intriguing natural products 2 featuring non-central chirality have been discovered over the and booming area of organocatalysis is heavily relying on non- years (Fig. 1).8,9 Not uncommonly, these secondary metabolites centrochiral architectures with some of the most prominent and exhibit interesting pharmacological activity. As expected for successful catalytic systems being based on the atropisomeric molecules interacting in a biological environment, the sense of binaphthyl backbone (Fig 2, bottom).16,17,18 chirality plays a crucial role in this regard and particularly in the In response to the growing demand for non-racemic, non- development and understanding of drugs, effects arising from centrochiral building blocks, in the past decades the development elements of axial or planar stereogenicity must not be 10,11 of synthetic methods towards axially, helically, and planar chiral overlooked. molecules in optically active form has attracted attention from Me various disciplines within organic chemistry. Here, also O Me H H biocatalysis – with its broad variety of catalytic systems, O 19,20 N excellent stereodiscrimination, and mild reaction conditions – H OMe O has been recognized as indispensable tool and found application H Br Me for the synthesis of many structural families featuring these non- 1, Okamurallene N classical chirogenicity elements. While the biocatalytic synthesis H OMe of smaller selections of non-centrochiral enantioenriched 2, Murrastifoline-F molecules has been covered to some extent as part of recent HO reviews,21,22,23 this report provides a full summary over all O OH O activites in the field over the past four decades. Introducing the MeO basic activation modes of the most frequently employed enzyme Me OMe classes, this review will highlight the power of biocatalysis – ranging from classical whole-cell systems to highly HO HO OMe MeOC contemporary tailor-made proteins – to effectively act on highly 3, Cavicularin MeO non-natural substrate structures in a synthetic fashion. O OH 4, Hypocrellin A Figure 1. Elements of non-central chirality in natural products. 2. Lipases and esterases as catalysts Among the many classes and subclasses of natural catalysts, A maybe even greater impact of axially, helically or planar the family of hydrolases has had by far most impact in the field chiral entities can be noted in the field of asymmetric catalysis. of synthetic chemistry. In particular, enzymes catalyzing Nowadays, many of the privileged ligand systems found in interconversions of carboxylic acid derivatives (esterases, lipases, stereoselective transition metal catalysis take advantage of the proteases) have gained credence within the organic community excellent properties of axially chiral binaphthyl core structure as over the years and are nowadays widely accepted as full-fledged controlling element.12,13 Likewise, with the appearance of synthetic tool. Natively located at the interface between aqueous ferrocenyl-based ligand families, inherently chiral organometallic and hydrophobic phases, the very high tolerance of lipases phosphines started to play a major role in transition metal against organic solvents represents one important factor for their catalysis not only in academia but also in the industrial process success. Although at first glance, ester hydrolysis is perhaps not design (Figure 2, top).14,15 In the same way, the relatively young regarded as synthetically challenging or exceedingly valuable transformation, the extraordinarily wide substrate scope together a) privileged ligands featuring non-central chirality with a rather promiscuous interpretation of their catalytic role – expanding to transesterifications, perhydrolysis, aminolysis et Me cetera – is turning the lipase/esterase enzymes into a very 24 PPh2 PCy powerful class of catalysts. The majority of hydrolases Fe 2 PPh2 PPh2 employed in preparative organic chemistry belongs to the serine PPh2 hydrolase superfamily. The common feature comprises a catalytic triad containing a nucleophilic serine that is typically 5, (R)-BINAP 6, (R,SP)-Josiphos Ph2P activated through a proton shuffle involving adjacent histidine and aspartate moieties. As depicted for the catalytic 7, (RP)-Phanephos transesterification (Scheme 1), the hydrogen-bonding arrangement within the triad substantially increases the serine's b) benchmark organocatalysts based on non-centrochiral architecture nucleophilicity, enabling attack of the hydroxy group at the ester Ar Ar substrate. The Ser105-bound first tetrahedral intermediate is Me further stabilized by hydrogen bond-donors (Gln106, Thr40) O O + forming the oxyanion hole. Elimination of alcohol R'OH leads to P N O OH Me the formation of an acyl-enzyme complex that is subsequently attacked by external nucleophiles (here: R''OH), resulting in a Ar Ar Me2N second tetrahedral intermediate. With the liberation of a new ester the catalytic cycle is terminated. From these considerations 8, (R)-TRIP N 9, Maruoka's phase transfer (Ar = 2,4,6-iPr Ph) Fe catalyst (Ar = 3,4,5-F Ph) 3 Ph Ph 3 it becomes apparent that enantiodiscrimination is potentially Ph Ph taking place both in the formation as well as in the decomposition Ph of the acyl-enzyme complex, thus allowing for stereoselective 10, ferrocene-DMAP-hybrid transformations (resolution or desymmetrization) involving either Figure 2. Features of axial and planar chirality in benchmark (pro)chiral carboxylate derivatives or (pro)chiral nucleophiles. systems of modern organo- and transition metal catalysis. 3 Asp187 Gln106 Ser O 105 O Thr40 O H O N O N H R OR'' His224 R OR' Asp187 Asp187 Gln106 Gln106 Ser105 Ser105 O O O O Thr40 Thr40 O O H O H O N N NH O NH O His R' His R 224 R'' 224 R' second tetrahedral first tetrahedral intermediate intermediate Asp187 Gln R''OH 106 R'OH Ser O 105 OH Thr40 H O O N N His224 R acyl-enzyme complex
Details
-
File Typepdf
-
Upload Time-
-
Content LanguagesEnglish
-
Upload UserAnonymous/Not logged-in
-
File Pages24 Page
-
File Size-