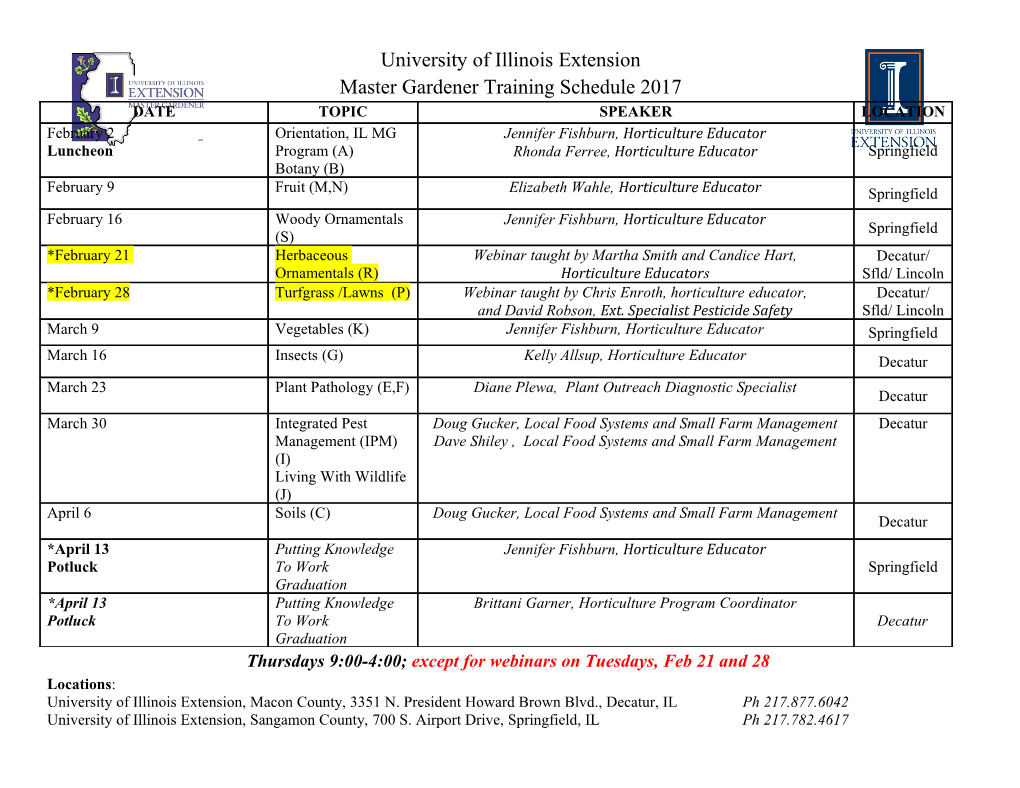
catalysts Review Merging Metallic Catalysts and Sonication: A Periodic Table Overview Claudia E. Domini 1,Mónica B. Álvarez 1, Gustavo F. Silbestri 1,*, Giancarlo Cravotto 2 and Pedro Cintas 3,* 1 Instituto de Química del Sur (INQUISUR), Departamento de Química, Universidad Nacional del Sur (UNS)-Consejo Nacional de Investigaciones Científicas y Técnicas (CONICET), Avenida Alem 1253, Bahía Blanca 8000, Argentina; [email protected] (C.E.D.); [email protected] (M.B.Á.) 2 Dipartimento di Scienza e Tecnologia del Farmaco, University of Turin, Via P. Giuria 9, 10125 Turin, Italy; [email protected] 3 Departamento de Química Orgánica e Inorgánica, Facultad de Ciencias-Universidad de Extremadura, and Instituto del Agua, Cambio Climático y Sostenibilidad (IACYS)-Unidad de Química Verde y Desarrollo Sostenible, Avda. de Elvas s/n, 06006 Badajoz, Spain * Correspondence: [email protected] (G.F.S.); [email protected] (P.C.); Tel.: +54-291-459-5101 (G.F.S.); Tel.: +34-924-289-300 (P.C.) Academic Editor: Keith Hohn Received: 8 March 2017; Accepted: 14 April 2017; Published: 19 April 2017 Abstract: This account summarizes and discusses recent examples in which the combination of ultrasonic waves and metal-based reagents, including metal nanoparticles, has proven to be a useful choice in synthetic planning. Not only does sonication often enhance the activity of the metal catalyst/reagent, but it also greatly enhances the synthetic transformation that can be conducted under milder conditions relative to conventional protocols. For the sake of clarity, we have adopted a structure according to the periodic-table elements or families, distinguishing between bulk metal reagents and nanoparticles, as well as the supported variations, thus illustrating the characteristics of the method under consideration in target synthesis. The coverage focuses essentially on the last decade, although the discussion also strikes a comparative balance between the more recent advancements and past literature. Keywords: metal catalysts; nanoparticles; organic synthesis; sonication; supported metal reagent/catalyst; ultrasound 1. Introduction The interaction of ultrasound with metals dates back to early sonochemistry, from the 1950s onwards, when some scientists discovered, rather by serendipity, that organometallic reagents and their subsequent coupling with organic compounds could be greatly improved by sonication in ultrasonic baths. The method clearly rivaled traditional procedures employed for metal reagents, as the ultrasonic protocol worked well without inert conditions and undried solvents. These pluses were initially associated to cleaning effects that removed the passivating coat of the otherwise unreactive zero-valent metals. There has been considerable progress since, and the reader is referred to past and recent major reviews that document in detail the mechanisms involved in activation of metal surfaces, notably by creating reactive sites by atomic dislocation and corrosion effects, as well as facile metalations and further reactions with an organic compound [1–5]. Of major significance in this context is the use of sonication in Barbier and Barbier-like reactions, where tedious and complex operations required to activate bulk metals are usually converted into simpler one-pot reactions [6,7]. Catalysts 2017, 7, 121; doi:10.3390/catal7040121 www.mdpi.com/journal/catalysts Catalysts 2017, 7, 121 2 of 29 The activation also applies to metal nanoparticles (NPs) [8–10], although their stability and reactivity may be substantially different from those of bulk metals, even though finely divided, and often require polymeric additives and/or deposition on inert supports, thus increasing the lifetimes of NPs in solution. Electron microscopy and X-ray diffraction reveal morphological surface changes induced by sonication on the crystal structure of metals such as Al, Cu, Zn, or Ag, which exhibit different hardness and natural roughness. As expected, cavitation plays a pivotal role, especially through mechanical effects generated by the collapse of microbubbles and the action of shock waves on a given surface. Both damage and deformation translate into structural reorganization of crystallites leading to small dimensional variations, weak grain cohesion, and atomic defects [11]. Chemical effects, especially surface oxidation induced by reactive species formed by substrate or solvent pyrolysis and released after bubble implosion, also contribute to structural changes of metal surfaces [12]. It is now well established that effects caused by cavitation can globally be rationalized by assuming a spherical model of bubbles, as microreactors, where three sonochemical reaction zones are identified [13]. The core or inner environment provides high local temperatures and pressures for volatile solutes capable of entering into the bubble and undergoing such intense conditions when it collapses. Given the extremely fast collapse and concomitant cooling rates (exceeding 1010 K per second), the structural reorganization and/or crystallization is highly hindered, thereby resulting in amorphous products. The interfacial region, between the core and the surrounding liquid, provides less intense conditions, yet high enough—ca. 1900 K—to bring about a chemical reaction. The interfacial region appears to be the prevalent zone where non-volatile substrates or those unable to enter into the bubble may react. Given the fast kinetics of collapse, the growth of the nuclei will also be considerable restricted and this may result in nanostructured or nanocrystalline materials depending on the temperature. The third zone comprising the bulk liquid at ambient conditions can also be the site for sonochemical reactions to occur, notably after releasing of reactive species generated by bubble collapse. However, the formation rate will also be lower [14]. The interplay between ultrasonic irradiation and metal activation can also be regarded within the green chemistry domain, even if some metal particles are inherently toxic materials and organic solvents cannot be completely avoided. However, these drawbacks are more than compensated for by the milder, faster, and more efficient processes run under sonication. Aqueous environments are often tolerated and new mechanistic pathways may afford functionalized derivatives not attained by thermal activation, thus switching conventional routes. In addition, it is worth pointing out that ultrasound along with other sustainable and enabling technologies provide sufficient activation to perform chemical reactions under solvent-free (i.e., neat reagents) and catalyst-free conditions [15]. This perspective is aimed at providing a good coverage of recent developments in the use of metal derivatives and metal NPs, generated and manipulated under ultrasound, hoping to be illustrative enough of the breadth and strength of the technique. The goals are focused on the capture of fundamental details that make ultrasound advantageous in a particular catalytic application. The preparation of micro- and nanomaterials with potential catalytic activity has grown almost exponentially. Since our analysis cannot be overlong, thus aiding readability and outlook of the applications presented, the article is largely restricted to synthetic strategies and/or specific applications, i.e., the sonochemical preparation of metal nanostructures or metal NPs without such detailed explorations cannot be treated, apart from a few exceptions in terms of discussion or comparative purposes. Accordingly, the authors hope to marry the properties of concision and representative cases, yet being informative and insightful. 2. Transition Metals and Supported Transition Metal Reagents The use of transition metals still represents the main domain of applications in synthesis and catalysis aided by ultrasound. Metal powders or metal alloys as well as other non-zero valent precursors, cheap metal oxides or metal halides for instance, are usually employed as precursors and Catalysts 2017, 7, 121 3 of 29 subjected to cavitation-induced treatments to generate active reagents and/or improve the synthetic transformation still further. To decrease metal loadings and improve the stability of metal particles, doping strategies on appropriate organic and inorganic supports are performed. Unfortunately, the nature of the resulting composite or soluble species is not always specified. The discussion Catalysts 2017, 7, 121 3 of 29 hereafterCatalysts identifies 2017, 7, 121 the material as stated in the original sources, while applications involving3 of NPs29 or nanostructurednature of the morphologies resulting composite will beor treatedsoluble species later. is not always specified. The discussion hereafter Late-transitionnatureidentifies of thethe resulting material metals, composite as copper stated or inin soluble particular,the origin speciesal provide issources, not always a while wide specified. rangeapplications ofThe synthetic discussion involving applications hereafter NPs or as Cu(I)identifiesnanostructured species arethe involvedmaterial morphologies as in stated efficient will bein andtreatedthe origin expeditious later.al sources, couplings. while applications Click reactions, involving generally NPs or based on copper-catalyzednanostructuredLate-transition morphologies [3+2]-cycloadditions metals, copper will be in treated particular, between later. provide azides a wide and range alkynes of synthetic (CuAAC) applications have met as many Late-transition metals, copper
Details
-
File Typepdf
-
Upload Time-
-
Content LanguagesEnglish
-
Upload UserAnonymous/Not logged-in
-
File Pages29 Page
-
File Size-