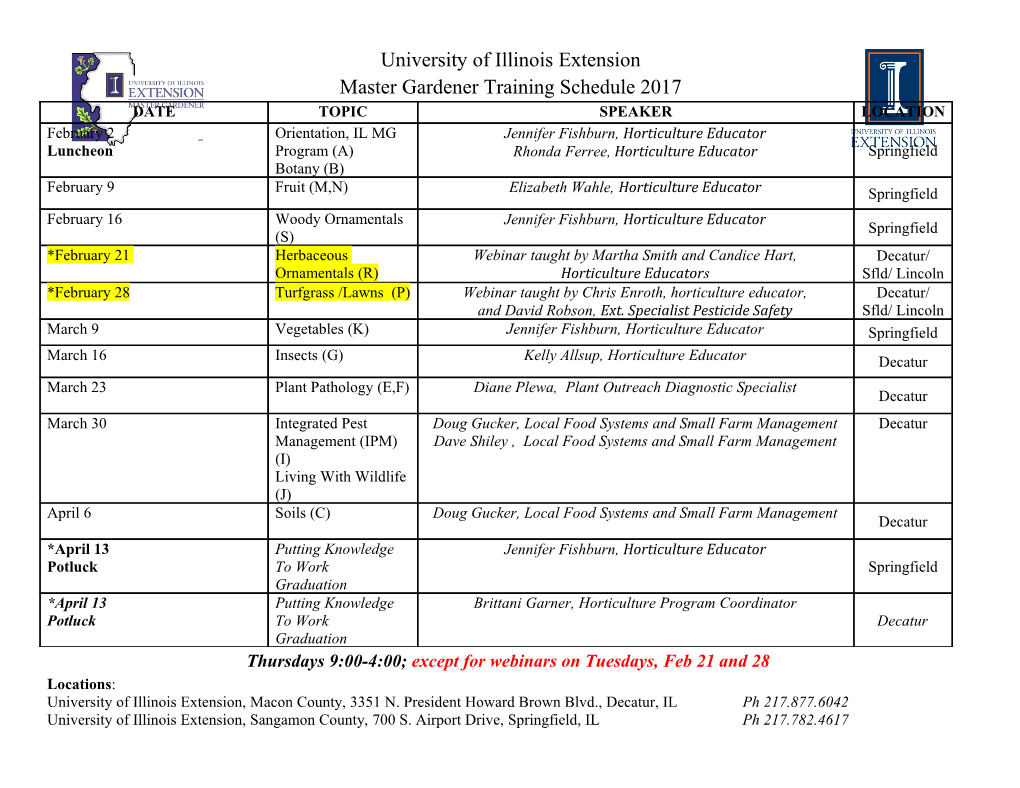
University of Tennessee, Knoxville TRACE: Tennessee Research and Creative Exchange Supervised Undergraduate Student Research Chancellor’s Honors Program Projects and Creative Work 5-2020 Measurements of fast alpha decays in the 100Sn region Ian Connor Cox University of Tennessee, Knoxville, [email protected] Follow this and additional works at: https://trace.tennessee.edu/utk_chanhonoproj Part of the Nuclear Commons Recommended Citation Cox, Ian Connor, "Measurements of fast alpha decays in the 100Sn region" (2020). Chancellor’s Honors Program Projects. https://trace.tennessee.edu/utk_chanhonoproj/2393 This Dissertation/Thesis is brought to you for free and open access by the Supervised Undergraduate Student Research and Creative Work at TRACE: Tennessee Research and Creative Exchange. It has been accepted for inclusion in Chancellor’s Honors Program Projects by an authorized administrator of TRACE: Tennessee Research and Creative Exchange. For more information, please contact [email protected]. Measurements of fast alpha decays in the 100Sn region A Thesis Presented for the Chancellor's Honors Program The University of Tennessee, Knoxville Ian Cox December 2019 Abstract The α decaying nuclei near 100Sn can provide a unique opportunity to study how the proximity to the N = Z line effects the α decay probability. It was hypothesized that these decays near 100Sn would have an increased preformation probability and thus higher α emission probability. 104Te is a special example where the effects manifest most drastically. The α decay of 104Te can provide useful insight into how proton-neutron interactions affect the formation of an α particle in the nucleus. The effects on α decay from particles interactions between particles in the same orbitals can be studied because of the self- conjugate and doubly magic nature of 100Sn. From previous experiments, the half-lives of nuclei in the region are found to be very short. In a recent experiment, the lifetime of 104Te was given an upper limit of 18 ns. For the half-life of 104Te to be accurately measured, a new detection technique will be implemented and a fast detector system has been built. Performance tests were carried out to prove the ability of a position-sensitive scintillator system, to measure the fast half-lives in the 108Xe to 100Sn α decay chain at an approved experiment at the RIKEN facility in Japan. Along with studying the 108Xe decay chain, the experiment will be able to study the lifetime of the first excited state of 101Sn populated in the α decay of 105Te. The detector system will have dual capabilities, with the inclusion of LaBr3 γ ray detectors, allowing for fast α-γ coincidence measurements to study excited states. ii Table of Contents 1 Introduction1 1.1 Motivation.....................................2 1.2 Previous Experimental Results..........................4 2 Overview of α decay models7 2.1 Phenomenological Models............................9 2.2 Microscopic Models................................ 11 3 Fast Detector System Development 13 3.1 Digitizer Testing................................. 13 3.2 Digital fast timing................................ 15 3.2.1 Fitting Analysis.............................. 16 3.2.2 Two Channel Timing........................... 17 3.3 Dynamic Range Testing............................. 21 4 Conclusions 23 Bibliography 25 iii List of Figures 1.1 A chart of nuclides showing the various decay modes from NNDC [13]. This highlights the different areas in which α decay has been observed.......3 1.2 Plotted data from Ref [10,2], where the preformation factor ( Wα) is on the vertical axis and the neutron number above the decay to a doubly magic nucleus is on the horizontal axis..........................5 2.1 A diagram for a particle tunneling through a potential barrier formed by a nucleus, from [6]. Where V is the potential curve and W is the energy of the particle.......................................7 2.2 Showing how the relationship of an α particle to the deformation of a nucleus effects the radius of the nucleus, similar to Ref [26]. Where β is the deformation parameter, taken from previous measurements........ 10 3.1 The setup used to created a delayed signal from the waveform generator to test the timing capabilities for the 500MHz digitizer.............. 14 3.2 A sample trace captured with a delayed pulse of 10 ns, generated from the set up shown in Figure 3.1 (left) along with the same pulse captured by a 4 GHz oscilloscope (right)................................. 14 3.3 PolyCFD algorithm on a plastic scintillator (left) and YAP scintillator (right). 16 3.4 Examples of the fitting algorithm for various scintillators, plastic (left), YAP (middle), YSO (right)............................... 17 3.5 Energy gating (left) used to measure the timing resolution (right) of 3 in. LaBr3 detector................................... 18 3.6 A phase-phase plot for two 3 inch LaBr3 detectors............... 19 iv 3.7 Demonstration of the walk in the timing resolution for plastic scintillators on PSPMT....................................... 20 3.8 The PSPMT demonstrates the ability to measure a smaller, secondary pulse as soon as 140 ns after a large inital pulse.................... 22 v Chapter 1 Introduction For every element, the number of neutrons can vary. From this, there are about 3000 known atomic nuclei where most are unstable. These radioactive nuclei can decay via various known modes. One of them is α decay, which occurs when the α particle tunnels through the Coulomb barrier created by the nucleus. Gamow first proposed to implement the tunneling model using quantum mechanics. In this model, the wavelike properties of particles allow for there to be a non-zero probability for the particle to travel from one region to another when separated by a finite potential barrier [4]. Tunneling is not possible in classical mechanics. In the α decay transition, the mass of the nucleus decreases by 4 atomic mass units (amu), due to an α particle being a 4He nucleus [9], as shown in the equation, AZ ! (A−4)(Z − 2) + 4He (1.1) With AZ being the parent nucleus and (A−4)(Z − 2) being the daughter nucleus. The energy in the decay is given by Qα, which is the difference between the rest mass of the parent nucleus, Mp, and the daughter nucleus, Md, and α particle, Mα [9]. Qα = Mp − Md − Mα (1.2) When the Qα values is positive, then the reaction is energetically favorable and occurs spontaneously [9]. 1 1.1 Motivation It has been known that some radioactive nuclei α decay since the early 20th century [18]. Early on, these decays were focused mainly on naturally occurring isotopes, such as Uranium. From these decays, Rutherford was able to determine that the α particle was simply the nucleus of the 4He atom by establishing the particle's charge to mass ratio [18]. Looking at a chart of nuclei, Figure 1.1, there are many nuclei which α decay. Due to the higher Qα values, α decay becomes more prominent in the heavy and superheavy elements. The higher Qα value indicates there is more energy in the decay, making the decay more energetically favorable. With so many nuclei decaying via α decay, it is important to be able to fully describe the mechanics behind this decay. Currently, there are evolved Gamow models that capture how the particle is able to escape the nucleus, but the question remains of how the four nucleons form within the nucleus. The α decay is similar to spontaneous fission, where Zelevinsky and Volya describe the process as a \junior-relative" [28]. In this case, the α particle is simply the smaller fragment from fission. Knowing the interactions that occur between protons and neutrons and how they influence clustering can also lead to better models for nuclear fission. The first studies of α decay studies examine heavy nuclei until Macfarlane and Siivola in 1965 found a new region of α decay near 100Sn [11]. This area in the chart of nuclei is interesting due to 100Sn having the same number of protons and neutrons, where both are magic numbers, N = Z = 50. Treating the daughter nucleus as a closed core, one can investigate how valence protons and neutrons interact when they are in the same states. Specifically, 104Te α decays to 100Sn allowing for insight into how the α particle is formed before decay. Currently, 212Po is considered to be a reference nucleus for α decay since its daughter nucleus, 208Pb, is doubly magic. Recently, an experiment was able to study the decay chain of 108Xe to 100Sn, allowing for experimental incorporation into theories regarding α decays in this region, but the few experimental points create large uncertainty [2]. An experiment to measure the α decays in the 100Sn region with a fast detector system has been approved at the RIKEN facility in Japan. The measurements would be focused on the 108Xe decay chain, but would also allow for measurements of similar decays in the 2 Figure 1.1: A chart of nuclides showing the various decay modes from NNDC [13]. This highlights the different areas in which α decay has been observed. area, such as the 109Xe decay chain. Previous measurements of α decays near 100Sn have found decays to excited states, which are then followed by γ emissions [10]. Using a fast detector system, these coincidence measurements can be made to determine the lifetime of the excited state. It is predicted that the M1 transition to the first excited state in 101Sn is retarded because it connects two orbitals with ∆L = 2 [3,1]. Both of these decay chains are detected through pileup signals in the detectors. This occurs when two decay events are detected sequentially, causing two pulses to be seen in the detector event. For this experiment to be successful, a fast detector system has to be designed and tested to measure the fast half-lives.
Details
-
File Typepdf
-
Upload Time-
-
Content LanguagesEnglish
-
Upload UserAnonymous/Not logged-in
-
File Pages36 Page
-
File Size-