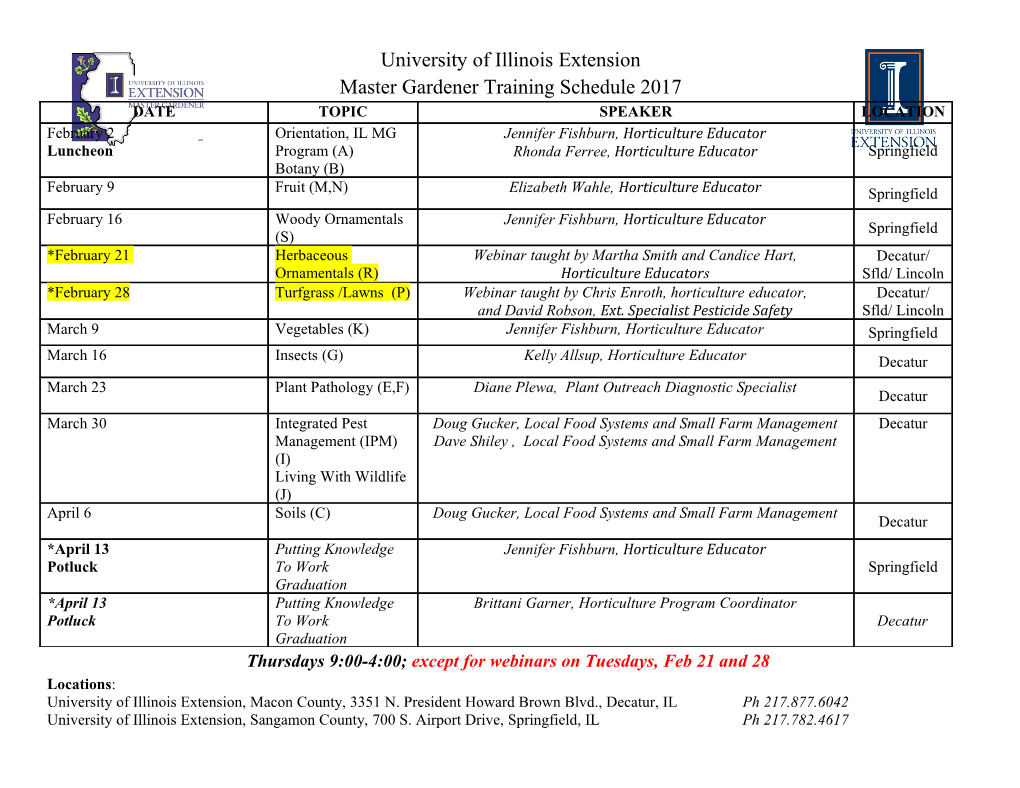
Washington University in St. Louis Washington University Open Scholarship Arts & Sciences Electronic Theses and Dissertations Arts & Sciences Spring 5-15-2017 Oxidative C-C Bond Formation Reactivity of High Valent Nickel and Palladium Complexes Michael Benjamin Watson Washington University in St. Louis Follow this and additional works at: https://openscholarship.wustl.edu/art_sci_etds Part of the Inorganic Chemistry Commons, and the Organic Chemistry Commons Recommended Citation Watson, Michael Benjamin, "Oxidative C-C Bond Formation Reactivity of High Valent Nickel and Palladium Complexes" (2017). Arts & Sciences Electronic Theses and Dissertations. 1152. https://openscholarship.wustl.edu/art_sci_etds/1152 This Dissertation is brought to you for free and open access by the Arts & Sciences at Washington University Open Scholarship. It has been accepted for inclusion in Arts & Sciences Electronic Theses and Dissertations by an authorized administrator of Washington University Open Scholarship. For more information, please contact [email protected]. WASHINGTON UNIVERSITY IN ST. LOUIS Department of Chemistry Dissertation Examination Committee: Liviu M. Mirica, Chair John R. Bleeke William E. Buhro Sophia E. Hayes Nigam P. Rath Oxidative C-C Bond Formation Reactivity of High Valent Nickel and Palladium Complexes. by Michael B. Watson A dissertation presented to The Graduate School of Washington University in partial fulfillment of the requirements for the degree of Doctor of Philosophy May 2017 St. Louis, Missouri © 2017, Michael B. Watson Table of Contents List of Figures v List of Schemes ix List of Tables x Acknowledgements xiii Abstract xv Chapter 1: Introduction to Palladium and Nickel Catalysis 1 1.1 Palladium Catalysis 2 1.2 Nickel Catalysis 4 1.3 Stabilization of High Valent Palladium and Nickel 6 1.4 References 10 Chapter 2: The Effect of Ligand Flexibility on the Stability of the Corresponding Pd(III) Complexes 13 2.1 Introduction 14 2.2 Experimental Section 16 2.2.1 Synthesis of (PyMe2tacn), L1 16 2.2.2 Synthesis of (Py2Metacn), L2 18 2.2.3 Synthesis of PdII Complexes 1-6 19 2.2.4 Physical Measurements 22 2.2.5 Computational Studies 25 2.3 Results and Discussion 25 2.3.1 Synthesis and Characterization of L1-L4 25 2.3.2 Syntheses and Characterization of PdII Complexes 26 2.3.3 X–ray Structure of Pd Complexes 26 2.3.4 Redox Properties 30 2.3.5 Chemical and Electrochemical Oxidation to Access the PdIII Oxidation State 32 2.3.6 Reactivity of [(L1)PdIII(Me)Cl]+ 34 2.3.7 DFT Calculations of PdIII Complexes 35 2.4 Conclusion 38 2.5 Acknowledgements 38 2.6 References 38 Chapter 3: Synthesis and Characterization of 1,4,7-Trimethyl-1,4,7-Triazacyclononane Supported Organometallic Ni(II), Ni(III), & Ni(IV) Complexes 43 3.1 Introduction 44 3.2 Experimental Section 45 3.2.1 Synthesis of Nickel Complexes 45 ii 3.2.2 Physical Measurements 46 3.2.3 Computational Studies 48 3.3 Results and Discussion 48 II 3.3.1 Synthesis and Characterization of (Me3tacn)Ni (CH2CMe2-o-C6H4), 1 48 III + 3.3.2 Synthesis and Characterization of [(Me3tacn)Ni (CH2CMe2-o-C6H4)] 50 IV 2+ 3.3.3 Synthesis and Characterization of [(Me3tacn)Ni (CH2CMe2-o-C6H4)(MeCN)] 53 3.3.4 DFT Calculations 55 3.4 Conclusion 60 3.5 Acknowledgements 61 3.6 References 61 Chapter 4: Reactivity of 1,4,7-Trimethyl-1,4,7-Triazacyclononane Supported Organometallic Ni(II), Ni(III), & Ni(IV) Complexes 65 4.1 Introduction 66 4.2 Experimental Section 67 4.2.1 Reactivity of 1 with Organic Halides 67 4.2.2 Aerobic Oxidation Studies of 1 68 4.2.3 Photolysis of 1, 2+, and 32+ 68 4.2.4 C-C/C-X bond formation reactivity of 2+ and 32+ 68 4.2.5 Physical Measurements 69 4.2.6 Computational Studies 69 4.3 Results and Discussion 70 4.3.1 Reactivity of 1 with Organic Halides 70 4.3.2 Aerobic Oxidation Studies of 1 72 4.3.3 Photolysis of 1, 2+, and 32+ 76 4.3.4 C-C/C-X Bond Formation Reactivity of 2+ and 32+ 78 4.4 Conclusion 80 4.5 Acknowledgements7 80 4.6 References 81 III Chapter 5: Synthesis and Characterization of (N4)Ni Cl2 Complexes and the Effects of Ligand Modification on Their Stability 85 5.1 Introduction 86 5.2 Experimental Section 87 5.2.1 Synthesis of tBuN4OMe Ligand 87 5.2.2 Synthesis of NiII/III Complexes 88 5.2.3 Physical Measurements 90 5.2.4 Computational Studies 91 5.3 Results and Discussion 91 5.3.1 Ligand Synthesis and Design 91 5.3.2 Synthesis and Characterization of NiII Complexes 93 iii 5.3.3 Synthesis and Characterization NiIIIComplexes 96 5.3.4 Transmetallation Reactions 100 5.3.5 Kumada Cross Coupling Reactivity 105 5.3.6 DFT Calculations on Complexes 1+-4+ 106 5.4 Conclusion 110 5.5 Acknowledgements 111 5.6 References 111 Chapter 6: Future Directions 114 6.1 Isolation of NiIV Species from Aerobic Oxidation 116 6.2 The Effect of Light on High Valent Ni Complexes 118 6.3 References 119 Appendix A: Select NMR Spectra of Ligands, Metal Complexes, and Reactivity 121 Appendix B: X-ray Diffraction Crystal Data of Transition Metal Complexes 151 Appendix C: Computational Data from DFT Calculations 237 iv List of Figures Figure 1.1: Examples of commonly used ligands. 4 Figure 1.2: Early examples of isolated high valent PdIII (left), NiIII (center), and NiIV 7 (right). R Figure 1.3: Different conformations of N4 and Me3tacn ligands. 8 Figure 1.4: High valent Ni and Pd complexes previously synthesized by our group 9 using RN4 and Me3tacn ligand. Figure 2.1: Structures of Multidentate Ligands Employed to Stabilize PdIII 15 Complexes. Figure 2.2: ORTEP view of 1 and 2 with 50% probability ellipsoids. All hydrogen 28 atoms, counter anions, and solvent molecules are omitted for clarity. Selective bond lengths: 1: Pd1‒N2 2.013(7); Pd1‒N1 2.020(2); Pd1‒N3 2.065(3); Pd1‒N2 2.140(4); Pd1‒Cl1 2.317(7); Pd‒N4 2.601(30); Pd1…N4 2.601(3). 2: Pd1‒C1 2.034(1); Pd1‒N1 2.044(1); Pd1‒N2 2.220(2); Pd1‒Cl1 2.296(4). Figure 2.3 ORTEP view of 5 with 50% probability ellipsoids. All hydrogen atoms, 29 counter anions, and solvent molecules are omitted for clarity. Selective bond lengths for 5: Pd1‒N1 2.012(3); Pd1‒N2 2.012(3); Pd1‒N3 2.018(3); Pd1‒Cl1 2.304(1); Pd1…N4 2.715(2). Figure 2.4: CV of 1 in MeCN at different scan rates. 30 Figure 2.5: CV of 2 in MeCN at different scan rates. 31 Figure 2.6: Absorption spectra of 1 (1 mM in MeCN) before (PdII) and after oxidation 33 to 1+ (PdIII). Figure 2.7: (a) EPR spectra of a 1+ and (b) 2+ in frozen MeCN-butyronitrile (1:3) 33 + glass. Simulation parameters: 1 , gx = 2.128; gy = 2.100; gz = 2.003; Azz + = 38 (IN = 1) and Azz = 35 (ICl = 3/2). 2 , gx = 2.211; gy = 2.055; gz = 2.038. Figure 2.8: Optimized geometry of 1+ (left). Selective bond lengths: Pd-N(1) 2.06156, 36 Pd-N(2) 2.12174, Pd-N(3) 2.10566, Pd-Cl(1) 2.47285, Pd-Cl(2) 2.68348. The spin density plot (right) corresponds to a 57% spin contribution from Pd. + Figure 2.9: Optimized geometries of three different geometries for 2 : (a) 2A; (b) 2B; 37 (c) 2C; Selective bond lengths for 2A: Pd-N(1) 2.232, Pd-N(2) 2.227, Pd- N(3) 2.389, Pd-Cl 2.446, Pd-Me 2.066; 2B: Pd-N(1) 2.143, Pd-N(2) 2.195, Pd-N(3) 2.204, Pd-N(4) 2.365, Pd-Cl 2.680, Pd-Me 2.066; 2C: Pd-N(1) 2.622, Pd-N(2) 2.275, Pd-N(3) 2.208, Pd-N(4) 2.291, Pd-Cl 2.680, Pd-Me 2.007. The spin density plot shown for 2A (top right) corresponds to a 67% spin contribution from Pd. Figure 3.1: CV of 1 in 0.1 M nBu4NPF6/MeCN (scan rate = 100 mV/s) (left). CVs of 50 1 in 0.1 M nBu4NPF6/THF, before and after addition of 10% v:v MeCN (scan rate = 100 mV/s). Note: the addition of MeCN leads to an v appreciable change in the redox event corresponding to the NiIV/III redox couple (right). Figure 3.2: Experimental (1:3 MeCN:PrCN, 77 K) and simulated EPR spectra of 2+. 52 The following parameters were used for the simulation: gx = 2.311, gy = 2.263, gz = 2.102 (A1N = 17.5 G). Inset: DFT-calculated spin density for 2+, shown as a 0.05 isodensity contour plot. Figure 3.3: Space filling model of 2+ as viewed from the bottom along the axial axis. 52 1 2+ Figure 3.4: H NMR spectrum and chemical shifts of 3 in CD3CN (500 MHz at -15 54 °C). Figure 3.5: UV-vis spectrum of 32+ in MeCN at room temperature. 55 Figure 3.6: DFT calculated Mulliken spin density of 2+ (shown with 0.005 isodensity 56 contour plot). From Mulliken atomic spin densities, all spin is located on the Ni Center. Figure 3.7: TD-DFT calculated (red) and experimental (black) UV-vis spectrum of 2+ 57 2+ (left) and 3 (right). 1 6 Figure 4.1: H NMR of 1 + O2 in 10% D2O/d -acetone at -30 °C (300 MHz).
Details
-
File Typepdf
-
Upload Time-
-
Content LanguagesEnglish
-
Upload UserAnonymous/Not logged-in
-
File Pages280 Page
-
File Size-