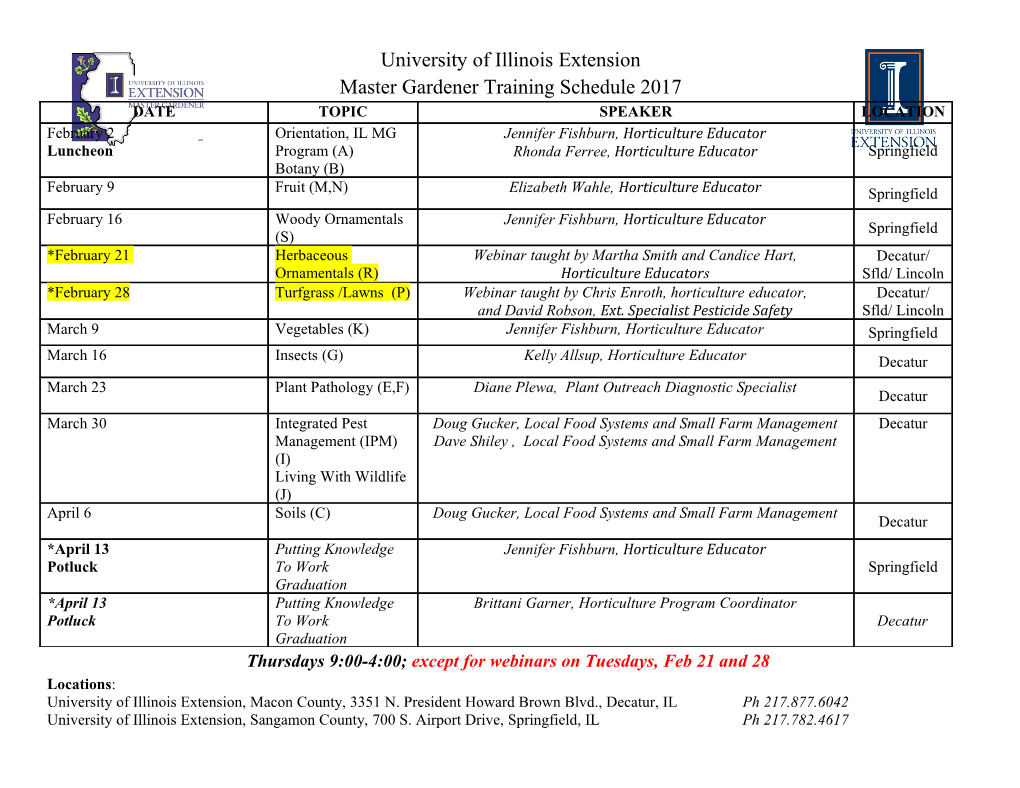
Opening New Windows Onto the Universe: Studies in Dark Matter, Dark Energy, and Gravitational Wave Sources DISSERTATION Presented in Partial Fulfillment of the Requirements for the Degree Doctor of Philosophy in the Graduate School of The Ohio State University By Matthew C. Digman, M.S. Graduate Program in Physics The Ohio State University 2020 Dissertation Committee: Christopher M. Hirata, Advisor John F. Beacom Eric Braaten Klaus Honscheid c Copyright by Matthew C. Digman 2020 Abstract The past two centuries have seen tremendous expansion in human understanding of the structure and contents of the universe. Yet, less than 5% of the mass of the universe is contained within particles predicted and explained by the modern Standard Model of particle physics. An even smaller fraction, less than 0:5%, of the mass of the universe is in familiar, tangible objects like planets and stars. With so much of the universe yet obscure to use, we must develop new techniques to observe what our eyes (and telescopes) cannot see directly. In this thesis, I present three papers I have written exploring new windows for observing the hidden aspects of the universe. In the first, presented in Chapter2,I explore theoretical constraints on models of strongly interacting dark matter. In the second, presented in Chapter3, I describe a formalism and code I developed to forecast the impact of long-wavelength density fluctuations on future weak lensing surveys. In the third, presented in Chapter4, I present a forecast for the number of white dwarf binaries that will be detected by both LISA and the Nancy Grace Roman Space Telescope. ii Acknowledgments I would like to thank the many people who have assisted me on the journey to my PhD. My high school physics teacher, Marshall Pauli, who helped to spark my interest in physics. My professors in the introductory physics curriculum at Dartmouth who helped motivate me to pursue physics as a major, including Marcello Gleiser, Lorenza Viola, and Robyn Millan. My undergraduate thesis advisor, Robert Caldwell, who ushered me into the field of cosmology, gravitational waves, and theoretical physics generally. My time at The Ohio State University and the Center for Cosmology and AstroParticle Physics has been a valuable period of growth for me as an individual and as a scientist. My advisor, Chris Hirata, has been a great influence and has helped me to grow immensely as a researcher and scientist. I will miss our conversations. Joe McEwen was a positive influence as a first year mentor and helped me get started in CCAPP. The older members of our research group, including Xiao Fang, Paulo Montero, and Daniel Martens were positive and supportive role models and sparked many valuable conversations. My past and current office mates, including Su-Jeong Lee, Benjamin Buck- man, Bianca Davis, Jenna Freudenberg, and Makana Silva have been the source of many fruitful and entertaining conversations. Chris Cappiello’s close collaboration was an invalu- able experience on our paper together. I have had many valuable conversations with the other graduate students in CCAPP, including Jahmour Givans, Gabriel Vasquez, Pawan Dhakal, Bei Zhou, Brian Clark, Jorge Torres Espinosa, and Kevin Fanning. The postdocs who passed through CCAPP during my time here have also been invaluable for conversation and mentorship, including Ashley Ross, Ami Choi, Niall MacCrann, Heidi Wu, Tim Linden, Jack Elvin-Poole, Johnny Greco, Katie Auchettl, and Anna Nierenberg. I am also grateful to my friends in physics at OSU outside of CCAPP, including Bryan Cardwell, Derek Everett, Daniel Heligman, Alexander Klepinger, Estefany Nunez, Andrew Piper, Bryan Reynolds, Srividya Suresh. Finally, thank you to Annika Peter and John Beacom who provided in- valuable guidance and education both through our paper together and my time at CCAPP generally. I’d like to thank my family for their invaluable support and inspiration throughout my education, career, and life, including my father Karl, my mother Laura, and my siblings iii Finn and Emily. I am also grateful to Emily Burland, who has been an invaluable source of support during my PhD. To everyone I mentioned above and others I surely forgot, you have helped me greatly through my PhD at OSU. This work would not have been possible without all of you. iv Vita 2015 . B.A., Dartmouth College 2017 . M.S., The Ohio State University Publications Forecasting Super-Sample Covariance in Future Weak Lensing Surveys with SuperSCRAM Digman, M. C., McEwen, J. E., Hirata, C. M. 2019, JCAP 10, 004 [arXiv:1904.12071] Not as Big as a Barn: Upper Bounds on Dark Matter-Nucleus Cross Sections Digman, M. C., Cappiello, C. V., Beacom, J. F., Hirata, C. M., Peter, A.G.H 2019, PRD 100, 063013 [arXiv:1907.10618] LISA Galactic Sources in the WFIRST Microlensing Survey Digman, M. C., Hirata, C. M. 2019, In Prep COSMIC Variance in Binary Population Synthesis Breivik, K., Coughlin, S. C., Zevin, M., Rodriguez, C. L., Kremer, K., Ye, C. S., Andrews, J. J., Kurkowski, M., Digman, M. C., Larson, S. L., Rasio, F. A. 2019, ApJ, Accepted [arXiv:1911.00903] Fields of Study Major Field: Physics v Table of Contents Page Abstract............................................ ii Acknowledgments....................................... iii Vita..............................................v List of Figures ....................................... ix List of Tables ........................................ xiii Chapters 1 Introduction1 1.1 Preamble ...................................... 1 1.2 Dark Matter..................................... 1 1.3 Dark Energy .................................... 2 1.4 Gravitational-Wave Detectors........................... 4 1.4.1 Background................................. 4 1.4.2 Detectors .................................. 4 1.5 Gravitational-Wave Sources............................ 9 1.6 Structure of Thesis................................. 10 2 Not as Big as a Barn: Upper Bounds on Dark Matter-Nucleus Cross Sections 11 2.1 Introduction..................................... 12 2.2 Dark Matter Scattering Theory.......................... 14 2.2.1 Overview of basic assumptions ...................... 15 2.2.2 Basic scattering theory........................... 16 2.2.3 Derivation of ‘model-independent’ scaling ................ 17 2.3 Contact interactions ................................ 20 2.3.1 Contact interaction with Born approximation.............. 20 2.3.2 Contact interaction with partial waves.................. 21 2.3.3 Attractive resonances ........................... 23 2.3.4 Beyond contact interactions........................ 24 2.4 Light Mediator ................................... 25 2.4.1 Momentum-transfer cross section..................... 26 2.4.2 Existing limits on light mediators..................... 26 2.5 Composite Dark Matter .............................. 27 2.6 Implications for Existing Constraints....................... 27 vi 2.6.1 Scaling constraints............................. 28 2.6.2 Detection ceilings.............................. 28 2.6.3 Dark matter-proton scattering constraints................ 29 2.6.4 Low-mass dark matter........................... 29 2.7 Conclusions..................................... 30 3 Forecasting Super-Sample Covariance in Future Weak Lensing Surveys with SuperSCRAM 38 3.1 Introduction..................................... 39 3.2 Overview ...................................... 41 3.2.1 Notation and Definitions.......................... 41 3.2.2 Workflow .................................. 41 3.2.3 Eigenvalue analysis............................. 44 3.3 Methods and Code Organization ......................... 45 3.3.1 Geometries ................................. 45 3.3.2 Short-Wavelength Observables ...................... 47 3.3.3 Long-Wavelength Observables....................... 47 3.3.4 Cosmological Parametrizations ...................... 49 3.3.5 Matter Power Spectrum.......................... 49 3.3.6 Fisher Matrix Manipulation........................ 49 3.3.7 Long-Wavelength Basis .......................... 51 3.4 Applications..................................... 51 3.4.1 Example Application: WFIRST+LSST ................. 51 3.4.2 Sensitivity to Survey Geometry...................... 53 3.4.3 Other Applications............................. 54 3.5 Conclusions..................................... 57 4 LISA Galactic Binaries in the Roman Microlensing Survey 59 4.1 Introduction..................................... 59 4.2 The surveys..................................... 60 4.2.1 Roman microlensing survey........................ 60 4.2.2 LISA..................................... 61 4.3 Methods....................................... 62 4.3.1 Binary population synthesis........................ 62 4.3.2 Tidal heating................................ 63 4.3.3 White dwarf atmospheres ......................... 63 4.3.4 Light curve generation........................... 64 4.3.5 Dust extinction............................... 64 4.3.6 Roman Exposures ............................. 65 4.3.7 Survey Strategy............................... 66 4.3.8 Chi-squared calculation .......................... 66 4.3.9 Selecting binaries.............................. 67 4.3.10 Trials factor................................. 67 4.3.11 Calculating detection efficiency...................... 68 4.4 Known systems................................... 68 4.5 Results........................................ 69 vii 4.6 Discussion...................................... 73 4.7 Conclusion ..................................... 73 5 Conclusion
Details
-
File Typepdf
-
Upload Time-
-
Content LanguagesEnglish
-
Upload UserAnonymous/Not logged-in
-
File Pages131 Page
-
File Size-