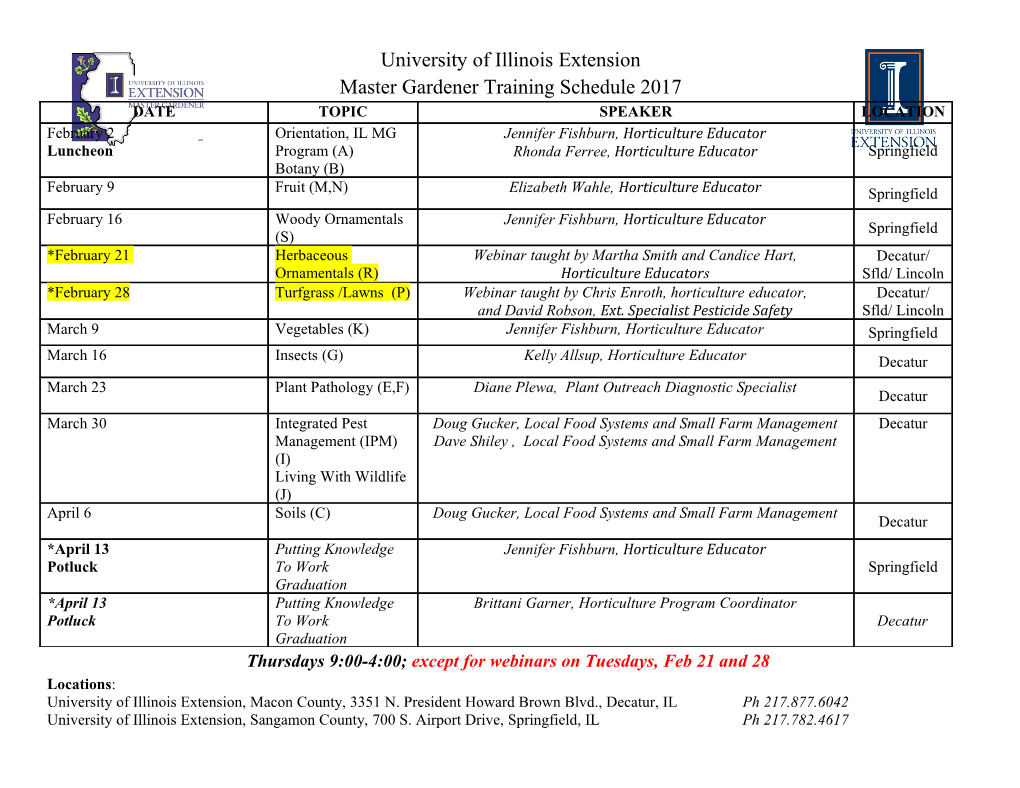
- 1 - Mating-type structure, evolution, and function in Euascomycetes R. Debuchy and B. G. Turgeon I. Introduction II. Sexual development in mycelial Euascomycetes III. Description of mating-type structure A. General organization of mating types 1. Loculoascomycetes a) Self-incompatible Loculoascomycetes b) Self-compatible Loculoascomycetes c) Asexual Loculoascomycetes 2. Sordariomycetes a) Self-incompatible Sordariomycetes b) Self-compatible Sordariomycetes c) Asexual Sordariomycetes 3. Leotiomycetes a) Self-incompatible Leotiomycetes b) Asexual Leotiomycetes 4. Eurotiomycetes a) Self-compatible Eurotiomycetes b) Asexual Eurotiomycetes B. Comparative organization of MAT context in Euascomycetes 1. MAT context in Loculoascomycetes 2. MAT context in Sordariomycetes and Eurotiomycetes C. Main features of mating-types genes and proteins 1. MAT1-1-1 2. MAT1-1-2 3. MAT1-1-3 and MAT1-2-1 IV. Evolution of mating types A. Phylogenetic analyses of mating type 1. Loculoascomycetes a) Cochliobolus b) Stemphylium 2. Sordariomycetes a) Gibberella b) Neurospora and Sordaria 3. Leotiomycetes: Aspergillus spp B. Functional analysis of MAT by heterologous expression 1. Conversion of a self-incompatible to a self-compatible strain 2. Conversion of a self-compatible to a self-incompatible species a) Loculoascomycete conversion b) Sordariomycete conversion V. Functions of mating-type genes A. Functions of the mating-type genes during fertilization 1. Regulatory functions of the mating-type genes 2. Target genes involved in fertilization - 2 - 3. A possible common scheme for fertilization control in Euascomycetes B. Functions of the mating-type genes during fruiting body development 1. C. heterostrophus 2. G. zeae 3. N. crassa 4. P. anserina VI. Conclusion Running title : mating types in Euascomycetes - 3 - I. Introduction Sexual reproduction requires sensing the environment to find a mate and mating engages a complex machinery for information exchange between individuals, cells, nuclei, chromosomes and genes. This very demanding process, called self-incompatibility or heterothallism in fungi, while conserved in general, has an intriguing history of modifications in this group of organisms, likely correlated with the wide range of natural environments. Self-incompatible individuals from the same species display different mating types. Individuals of identical mating type are defined, primarily, as individuals that cannot mate with each other, but can mate with individuals, from another group, that display a different mating type. This phenotypic complexity can be disentangled by genetics, which permits one to establish how many loci control mating type. To date, all self-incompatible Euascomycetes have been found to harbour only one locus, (MAT), which contains one or the other of two possible forms of genetic information establishing mating type. An alternative mating strategy, called self-compatibility is less demanding than self-incompatibility. Homokaryotic cultures of self-incompatible fungi can undergo self-mating. These fungi (also known as homothallic fungi) nevertheless perform all steps of meiosis. A special case of self- compatibility (also known as pseudohomothallism or secondary homothallism) has been described for a few genera. These isolates can be resolved into homokaryotic self- incompatible cultures, indicating that isolates are heterokaryons, carrying nuclei of opposite mating type. A survey of 10,596 ascomycetes showed that 55 % of them may reproduce sexually, but evidence of mating is lacking for 45 % of the 10,596 species (Reynolds and Taylor 1993). W hether or not these apparently asexual species ever undergo sexual reproduction or have cryptic or rare sex is often debated. A decade ago, when mating type genes were first identified, finding mating-type genes in asexual fungi was a novel discovery (first reported by (Sharon et al. 1996)), but is now commonplace. W hatever the reason for lack of a sexual stage (cryptic sexuality, mutations in key genes) absence of the sexual reproductive machinery is not the answer. This finding supports the notion that the asexual life style arises from sexual progenitors. This chapter emphasizes new developments in our knowledge of mating-type structure in self-compatible, self-incompatible, and asexual Euascomycetes. It presents a possible scenario for mating evolution among the three different classes of fungi, as deduced from the mating-type structures, and considers functions attributed to the mating-type genes. Space limitations excluded consideration of several fields that the reader can find in other reviews: e.g., review with a focus on the genetic aspects of unstable mating types (Perkins 1987); review with a focus on theoretical aspects of mating type (Metzenberg 1990); review with a focus on mating type functions (Metzenberg and Glass 1990); review with a focus on vegetative incompatibility (Glass and Kuldau 1992); review with a focus on genetic aspects of mating systems (Coppin et al. 1997); review with a focus on physiology of intercellular recognition (Bistis 1998); review with a focus on evolution and pathology (Turgeon 1998); review with a focus on mating types and biotechnology (Pöggeler 2001); review with a focus on commonalities between Ascomycetes and Basidiomycetes (Casselton 2002); reviews with a general scope (Glass and Lorimer 1991; Glass and Nelson 1994; Kronstad and Staben 1997; Hiscock and Kües 1999; Shiu and Glass 2000; Souza et al. 2003). II. Sexual development in mycelial ascomycetes The life cycle of Euascomycetes that are used as model systems, such as Podospora anserina and Gibberella zeae, is presented in Fig. 1. Self-incompatible homokaryotic strains of either mating type produce female reproductive structures, the ascogonia, and male cells, the spermatia. The spermatia are true differentiated sexual cells unable to germinate, in contrast to - 4 - conidia. Fertilization occurs between an ascogonium of one mating type and a donor cell of opposite mating type. The donor cells may be spermatia, microconidia, macroconidia or hyphae. Ascogonia are topped by a specialized hypha, the trichogyne, which is attracted by the donor cell of opposite mating type and eventually fuses with it (Bistis 1998). Fertilization is followed by the migration of the male nucleus down the trichogyne towards the body of the female organ. During its migration, the male nucleus passes by female nuclei and when present in the body of the female organ, undergo a series of mitotic divisions resulting in the formation of plurinucleate cells containing nuclei of opposite mating types. Successful production of offspring depends on internuclear recognition, a process associated with cellularization whereby two nuclei, one nucleus of each mating type, migrate to form specialized dikaryotic hyphae. These hyphae, called ascogenous hyphae, maintain a strict ratio of 1:1 of each parental nucleus. Eventually, the tip of this type of hypha differentiates into a crozier, which consists of two uninucleate cells and one dikaryotic cell. Nuclei fuse and meiosis ensues immediately while the cell is elongating to form the ascus. After a post- meiotic mitosis, ascospores are delineated inside the ascus and eventually projected outside the fruiting body (see Chap. 20, this Vol.). In self-compatible species, the male nucleus required for fertilization is assumed to be provided to the ascogonium by its basal cell, but outcrossing indicates that external cells can occasionally be the source of a male nucleus. Variations to this description can be found in Euascomycetes, but the formation of a plurinucleate cell after fertilization and cellularization associated with internuclear recognition leading to the formation of ascogenous hyphae seems to be the common scheme. III. Description of mating-type structure A. General organization of mating types In all self-incompatible filamentous ascomycetes examined to date, the mating-type locus contains one of two different sequences occupying the same chromosomal locus in their genome. The extent of dissimilarity ranges from 1 kb to almost 6 kb. Metzenberg and Glass used the word idiomorph to denote these large sequences not obviously related by structure or common descent (Metzenberg and Glass 1990). The analysis of self-compatible species indicates that, in most cases, both mating types are contained in the haploid genome, usually tightly linked, but sometimes not, and thus these loci cannot be called idiomorphs, since there is no second strain of opposite mating type. W e retain the use of mating type, instead of idiomorph, for self-compatible species. A standardized nomenclature for idiomorphs and mating-type genes has been proposed, and, in most cases adopted, to cope with the rapidly growing number of described mating-type loci from various species (Turgeon and Yoder 2000). This standardized nomenclature is based on the constant presence in one idiomorph of an ORF encoding a protein with a motif called the α1 domain, found initially in the MATα1 transcription factor of Saccharomyces cerevisiae. This idiomorph is called MAT1-1 and the corresponding gene MAT1-1-1. The other idiomorph is characterized by the constant presence of an ORF encoding a protein with a DNA-binding domain of the high mobility group (HMG) type and is called MAT1-2. The corresponding gene is called MAT1-2-1. The number assigned to any particular gene within an idiomorph corresponds to that of its homolog in other fungi that have already characterized MAT genes. If there is no apparent homolog,
Details
-
File Typepdf
-
Upload Time-
-
Content LanguagesEnglish
-
Upload UserAnonymous/Not logged-in
-
File Pages46 Page
-
File Size-