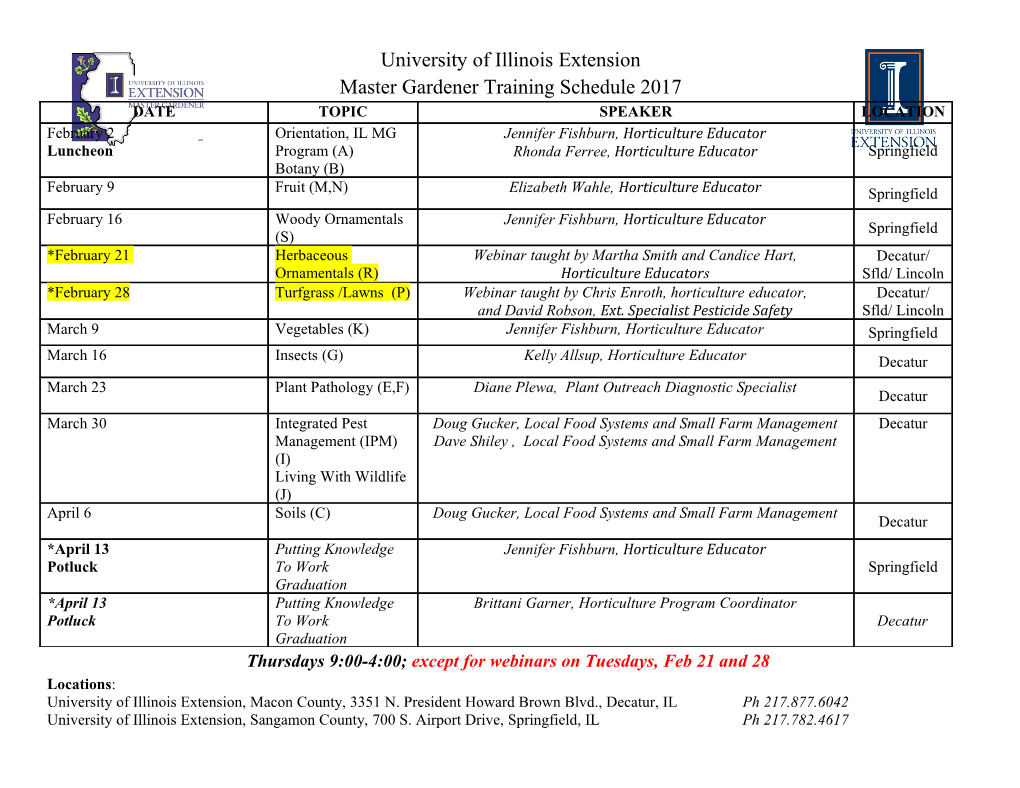
Unveiling Potent Photooxidation Behavior of Catalytic Photoreductants Karina Targos,‡ Oliver P. Williams,‡ and Zachary K. Wickens* Department of Chemistry, University of Wisconsin–Madison, Madison, Wisconsin 53706, United States. Supporting Information Placeholder 4 ABSTRACT: We describe a photocatalytic system that intersystem crossing. Despite tremendous effort in 4,5 reveals latent photooxidant behavior from one of the most photoredox catalyst design, excited state potentials reducing conventional photoredox catalysts, N- beyond roughly –2 and +2 V vs SCE remain difficult to 6 phenylphenothiazine (PTH). This aerobic photochemical achieve using conventional photocatalysis (Figure 1A). reaction engages difficult to oxidize feedstocks, such as Unfortunately, this redox window excludes numerous benzene, in C(sp2)–N coupling reactions through direct abundant hydrocarbon feedstocks from facile 7 oxidation. Mechanistic studies are consistent with activation photoinduced electron transfer. of PTH via photooxidation and that Lewis acid co-catalysts To overcome the energetic limitations intrinsic to scavenge inhibitors formed upon catalyst activation. conventional photoredox catalysis, König and coworkers recently designed a photocatalytic system that drives challenging reductive SET events using the energy of two photons rather than one. This consecutive photoninduced Reactions driven by single electron transfer (SET) are electron transfer (conPET)8 strategy relies on a catalytic pervasive in organic chemistry. Consequently, new photooxidant and sacrificial reductant that, upon strategies to induce redox events are poised to profoundly irradiation, result in a potent radical anion photoreductant impact synthetic chemistry.1 Photoredox catalysis has (Figure 1B). Despite its mechanistic complexity, this unlocked a broad range of attractive new transformations approach is practical and operationally simple; it leverages through conversion of energy from readily accessible LEDs inexpensive and safe LEDs to accomplish reactions that into chemical redox potential.2 However, only a portion of otherwise require UV photoreactors or harsh chemical this energy3 can be harnessed due to inevitable energy reductants. Following proof-of-concept aryl halide losses from vibrational relaxation, internal conversion, and reductions,8,9 this approach has enabled photochemical Table 1. Survey of oxidants and photoreductants.a–d CO2Et alternatives to alkali metal reductants in reactions such as CO2Et 10 11 [catalyst] Birch reductions and sulfonamide cleavage. + N HN N N LED irradiation In contrast to the progress in photoreductions, 1 [oxidant] 2 oxidations driven by the consumption of multiple photons have remained elusive. We suspect that this is the 100 Ar2 consequence of two inextricable challenges: (1) the N N N catalyst must be a competent photocatalyst in both the 75 S Ar1 O Ar1 N 2a,8b closed shell and radical cation states and (2) the Ar2 PTH PC-1 PC-2 terminal oxidant must efficiently activate the catalyst but 50 not otherwise interfere with the reaction (Figure 1C).12 yield (%) Given the difficulty applying multiple photons towards a 25 challenging SET oxidation, photoredox reactions initiated by SET oxidation are typically limited to electron-rich 0 13 PhI PhBr CH NOPFO O PhI PhBr CH NOPFO O PhI PhBr CH NOPFO O hydrocarbon substrates. 2 2 d 2 2 d 2 2 d 2 Br 2 Br 2 Br Our group is investigating diverse strategies to expand 2 6 2 6 2 6 a 14 Reactions were conducted on a 0.05 mmol scale in 1:1 MeCN:PhH for the redox potentials accessible via photoredox catalysis. 1 24h, unless noted otherwise, using 2 equiv oxidant or 1 atm O2. Ar = 4- We questioned whether conventional photoreductants, biphenyl. Ar2 = 2-napthyl. bPTH irradiated using 390 nm Kessil lamp. cPC- 1 and PC-2 irradiated using Tuna Blue Kessil lamp. d1:1 TFE:PhH. which typically possess persistent radical cation states, radical cation without additional energy from light, could be repurposed as strong photooxidants using a 24 NOPF6, provided low yield of benzene oxidation conPET strategy.15 We hypothesized photochemical products using all three catalysts. However, under one conditions designed to drive these photocatalysts towards atmosphere of O2, PTH promoted oxidative coupling in their oxidized congeners could reveal oxidation behavior. promising yield (14%). Given the enhanced stability of To probe this hypothesis, we targeted cyclic triarylamine radical cations in fluorinated alcohol solvents,25 we photoreductants. These are a well-established and modular substituted MeCN for trifluoroethanol (TFE). This family of neutral photoreductants6d,16 and isolated reports resulted in a modest increase in reaction yield with PTH suggest their radical cation congeners could exhibit but only traces of product with the other two catalysts. Of photochemical activity.17 We selected the Nicewicz-type13e note, while most photoredox catalysts undergo rapid oxidative coupling of arenes and N-heterocyclic intersystem crossing to a long-lived triplet,2a,5c PTH is a nucleophiles as a model reaction. This synthetically singlet excited state reductant.26 This property circumvents valuable transformation is representative of the general photocatalyst deactivation by triplet-triplet annihilation challenges in oxidative photoredox catalysis; it has been with O2. predominantly limited to electron-rich arene substrates, such as anisole derivatives.18 Difficult to oxidize arene We suspected that BET between PTH radical cation and 27 substrates, such as benzene, mandate high energy UV light superoxide might attenuate reactivity under these 28 (UVB or shorter),6a strong ground state oxidants (e.g. conditions (Figure 2). Indeed, when synthetically prepared DDQ) that absorb visible light,19 or more technically PTH radical cation is treated with KO2, we observe a rapid 1 complex electrophotocatalytic approaches.20,21 Thus, color change and reformation of PTH by H-NMR. Given beyond providing a proof-of-concept, we envisioned that that superoxide generation is inextricable from aerobic promoting this transformation using a stable photocatalyst with only inexpensive LEDs would be a synthetically useful complement to existing methods. First, we examined three distinct photoreductants6d,22 and a range of oxidants for activity in the oxidative coupling 23 of benzene (Eox = 2.5 V vs. SCE) and pyrazole 1 (Table 1). We initially aimed to generate the catalyst radical cation congener via photoreduction of reagents that undergo irreversible decomposition after SET to avoid catalyst deactivation via back electron transfer (BET). Excitingly, these data revealed that N-phenylphenothiazine (PTH), the most reducing photocatalyst of the series, could promote this challenging oxidative coupling in low yield using organohalides as the oxidant. A sufficiently strong oxidant to oxidize each catalyst to the corresponding 2 catalyst activation, this observation could account for the of the additive, suggesting a co-catalytic role for LiClO4 rather modest reactivity of this catalytic system (Table 2, entry 1). than it purely sequestrating stoichiometric byproducts (entry As expected, addition of 5 mol % KO2 to the reaction mixture 4). In principle, the lithium co-catalyst could mitigate BET by completely suppressed product formation (entry 2). We promoting superoxide disproportionation.29 Consistent with hypothesized that additives capable of sequestering or this proposed Lewis acidic role, alkylammonium salts had no scavenging this inhibitor would enhance the observed impact on the reaction (entry 5); whereas, other Lewis acidic reactivity. Guided by this model, we found addition of one lithium salts retained the catalytic effect (entries 6 and 7). equivalent of an inexpensive, redox innocent Lewis acid, Final tuning of the reaction parameters revealed adjusting the LiClO4, dramatically improved the yield of oxidative coupling solvent mixture to include a small amount of product (entry 3). Reduction of the LiClO4 loading to a hexafluoroisopropanol (HFIP) delivered the desired product substoichiometric quantity (20 mol %) retained the benefits in 89% yield (entry 8). Substitution of PTH with other Table 2. Reaction optimizationa triarylamine photoreductants or a classic metal-based CO2Et CO2Et PTH (5 mol %) photoreductant, Ir(ppy)3, resulted in only trace yield of [additive] + N oxidation product under these otherwise optimal conditions HN N N 390 nm LED (entries 9–11). 1 O2 (1 atm) 2 Having identified a promising catalytic system, we entry additive yield (%) examined the scope of this new process (Table 3). Pyrazole 1 none 31 nucleophiles bearing a range of electron-withdrawing 2 KO2 (5 mol %) <2 moieties, including ketones (3), aldehydes (4), nitriles (5), 3 LiClO4 (1 equiv) 86 and trifluoromethyl groups (6) were oxidatively coupled to 4 LiClO4 (20 mol %) 73 benzene. Halogenated pyrazoles (7 and 8) were also 5 n-Bu4ClO4 (20 mol %) 29 productively coupled despite the fact that PTH is a potent 6 LiPF6 (20 mol %) 64 photoreductant. Prior approaches capable of oxidizing 7 LiOTf (20 mol %) 56 benzene have not been readily amenable to the arylation of b 30 8 LiClO4 (20 mol %) 89 neutral heterocyclic substrates. In contrast, we observed b,c 9 PC-1 (5 mol %), LiClO4 (20 mol %) <2 coupling of both parent pyrazole (9) and even an electron- b,c 10 PC-2 (5 mol %), LiClO4 (20 mol %) <2 rich analog (10), albeit in diminished yield relative to the b,c 11 Ir(ppy)3 (1 mol %), LiClO4 (20 mol %) <2 electron deficient heterocyclic coupling partners.
Details
-
File Typepdf
-
Upload Time-
-
Content LanguagesEnglish
-
Upload UserAnonymous/Not logged-in
-
File Pages8 Page
-
File Size-