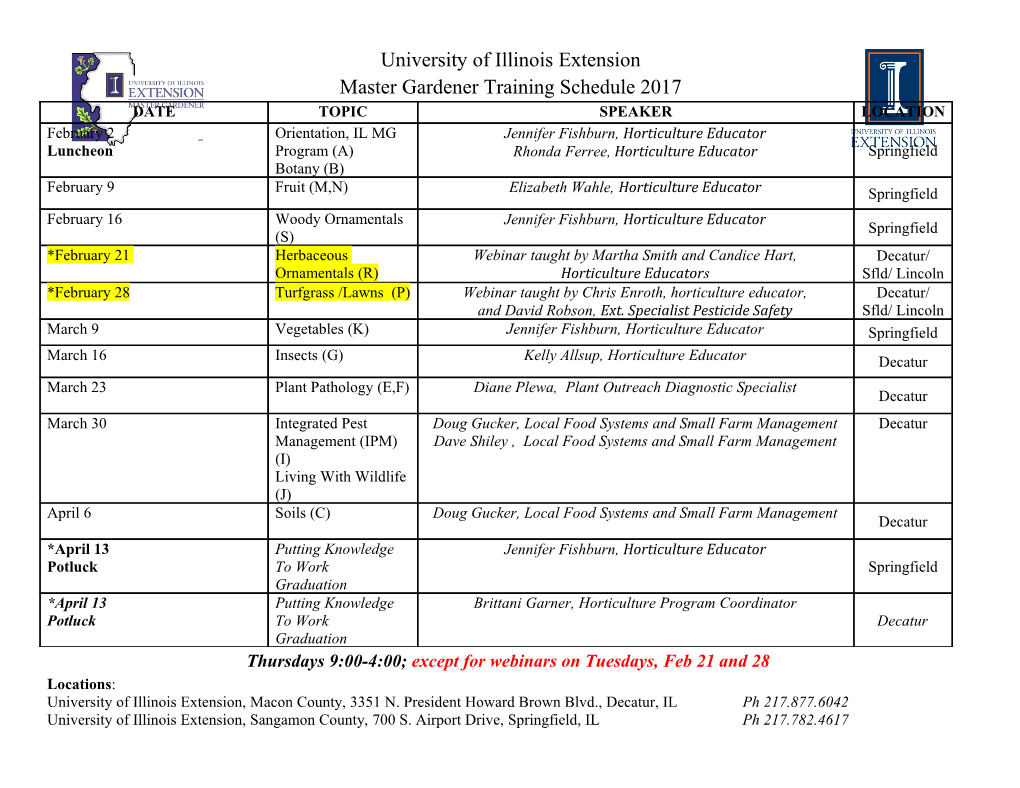
applied sciences Article A Theoretical Model for the Triple Phase Boundary of Solid Oxide Fuel Cell Electrospun Electrodes Wei Kong *, Mengtong Zhang, Zhen Han and Qiang Zhang * School of Energy and Power, Jiangsu University of Science and Technology, Zhenjiang 212003, Jiangsu, China; [email protected] (M.Z.); [email protected] (Z.H.) * Correspondence: [email protected] (W.K.); [email protected] (Q.Z.); Tel.: +86-511-84417398 (W.K.); Fax: +86-511-84404433 (W.K.) Received: 31 December 2018; Accepted: 28 January 2019; Published: 31 January 2019 Featured Application: Solid oxide fuel cells (SOFC) are one of the power-generated devices that have received much attention over the last decade due to advantages, such as a high efficiency, quietness, multiple fuels, and the inexpensive catalyst nature. To improve the performance of SOFC, the electrospun electrode is introduced to the SOFC, which can be achieved by impregnating nanoscale particles on the backbone surface of fibers. In this paper, a theoretical model was developed for the electrospun electrode. This model captures the key geometric parameters and their interrelationships, which can be used to design the microstructure parameters, such as the particle radius, fiber radius, and impregnation loading. Abstract: Electrospinning is a new state-of-the-art technology for the preparation of electrodes for solid oxide fuel cells (SOFC). Electrodes fabricated by this method have been proven to have an experimentally superior performance compared with traditional electrodes. However, the lack of a theoretic model for electrospun electrodes limits the understanding of their benefits and the optimization of their design. Based on the microstructure of electrospun electrodes and the percolation threshold, a theoretical model of electrospun electrodes is proposed in this study. Electrospun electrodes are compared to fibers with surfaces that were coated with impregnated particles. This model captures the key geometric parameters and their interrelationship, which are required to derive explicit expressions of the key electrode parameters. Furthermore, the length of the triple phase boundary (TPB) of the electrospun electrode is calculated based on this model. Finally, the effects of particle radius, fiber radius, and impregnation loading are studied. The theory model of the electrospun electrode TPB proposed in this study contributes to the optimization design of SOFC electrospun electrode. Keywords: solid oxide fuel cells; electrospinning; triple phase boundary; electrode 1. Introduction Solid oxide fuel cells (SOFC) have received much attention over the last decade due to advantages, such as a high efficiency, quietness, multiple fuels, and the inexpensive catalyst nature [1–4]. One of the major challenges related to SOFCs is the short lifetime introduced by the high operating temperature. The decrease of the working temperature is an effective method to increase the lifespan of SOFC. Therefore, lower or intermediate temperature SOFC have been extensively studied in recent years [5,6]. The electrochemical reactions in the SOFC electrode only take place at the so-called triple phase boundary (TPB), where oxygen ions, electrons, and gaseous species cohere. The reaction activity of the TPB sharply decreases with decreasing temperature. Therefore, it is significant for lower or intermediate temperature SOFC to have a long TPB. Appl. Sci. 2019, 9, 493; doi:10.3390/app9030493 www.mdpi.com/journal/applsci Appl. Sci. 2019, 9, 493 2 of 11 The TPB length is a critical geometric parameter of the electrode and dominates the SOFC and electrode performance [7,8]. Meanwhile, the TPB itself is strictly associated with the electrode micromorphology [9,10]. To further improve the electrode performance, many studies focused on deriving an optimal micromorphology from various fabricating processes, such as screen-printing [11,12], freeze-casting/phase inversion [13,14], co-precipitation [15], impregnation/infiltration [16,17], and electrospinning [18,19]. Several types of electrodes with distinct microstructures have been successfully prepared. Conventional electrodes (CE), which are fabricated by sintering composite powders, have been widely and deeply studied both experimentally and theoretically. The TPB length of CE can be extracted from the detailed microstructure obtained, by focused ion beam-scanning electron microscopy (FIB-SEM) [20,21] or X-ray diffraction [22]. Theoretical models for TPB calculation can be used to systematically study the impacts of the electrode properties, including the particle size, porosity, and component fraction on the TPB length. Among those models, the random sphere packing model has become popular [23–25]. However, to improve the electrode performance or lower the operation temperature, limitations of the CE, such as the incompatible thermal expansion coefficient of the anode (e.g., Ni and yttria-stabilized zirconia (YSZ)) and high polarization loss of the cathode [26–28], have to be overcome. To address those problems, a nanostructured electrode can be introduced to the SOFC, which can be achieved by impregnating nanoscale particles on the backbone surface of another phase [16,29]. The nanostructure of the impregnated electrode (IE) leads to a tremendous enhancement of the TPB length and the impregnation phase volume fraction (e.g., Ni) is much lower than that of the CE [30]. From a phenomenological viewpoint, there are two distinct IE constituents: backbone and coated impregnation nanoparticles. These features constitute the basis of theoretical models because the IE is modeled in many cases as a sphere-packed backbone with a surface that is coated with nanospheres of another phase [26,30]. Because the TPB length of the nanostructured electrode is directly related to the total backbone surface area [31], it is logical to explore new frameworks with increased surface areas to improve the electrode performance. The basic backbone formed by many one-dimensional nanostructures (e.g., nanofibers) possesses a high surface area and porosity [32], which implies a novel backbone structure. Because electrospinning is a simple and effective method to produce uniform one-dimensional nanofibers with a high specific area and porosity, it has become a new state-of-the-art technology for the preparation of electrodes for SOFCs [33]. Li et al. [34] studied fibrous Ni-coated YSZ anodes, where the YSZ nanofibers were prepared via electrospinning and then electrolessly plated with a layer of Ni. Their results suggested that the Ni loading is only 30 wt.%, that is, lower than that of CE. Two anodes with the same Ni fraction were prepared by electrospinning and power processing, respectively. The test results showed that the peak power density of the cell with the electrospun anode is twice that obtained using the power-processing anode, which was attributed to the longer TPB of the former anode. Zhao et al. [33] compared La0.8Sr0.2Co0.2Fe0.8O3−δ (LSCF) nanorod/Ce0.8Gd0.2O1.9 (GDC) nanoparticle composite cathode with LSCF/GDC nanoparticle cathode. The polarization resistance of the former is five times smaller than that of the latter. Further studies indicated that the cathode performance is better when the diameter of the nanofibers is sufficiently small [33,35]. Other studies [36,37] confirmed the significant influence of the microstructure and intrinsic properties of the basic components on the cathode performance. Although the CE and IE have been widely studied, the electrospun electrode (EE) morphology differs from that of the CE or IE. The models and results for the CE and IE cannot be directly applied to the EE. The 3D reconstruction via FIB-SEM or X-ray diffraction is a powerful tool to quantitatively analyze the geometric properties of CEs. However, those methods cannot be used to reconstruct the impregnated microstructure due to the resolution gap [31]. Therefore, although the experimental activities are strong, as aforementioned, most experiments focused on the preparation and testing of the macro-performance; quantitative analyses of the geometric properties are rare. Contrary to in-depth Appl. Sci. 2019, 9, 493 3 of 11 experimental studies, there is little theoretical research, to the best of our knowledge. The work of Enrico, A. and Costamagna, P [38] was developed specifically for LSCF fibers. Being LSCF a MIEC (mixed ionic electronic conductor, embedding both electronic and ionic conduction simultaneously), a number of infiltrated particles below the percolation threshold were considered. Conversely, in the present work, the fibers are considered to be a pure ionic conductor, and percolation of the infiltrated electronic conducting particles is a mandatory feature for the electrochemical reaction to expand into the electrode bulk, i.e. for the infiltration/fiber interface to contribute to the TPB. In this paper, based on the microstructure of EE reported in literatures [18,19] and the percolation threshold, a theoretical model was developed for the EE by comparing the EE to fibers with surfaces that were coated with impregnated particles. This model captures the key geometric parameters and their interrelationship, which can be used to derive explicit expressions of several key electrode parameters. Furthermore, the TPB length of the EE was calculated based on this model. Finally, the effects of the particle radius, fiber radius, and impregnation loading were systematically studied. 2. Theoretical Model Generally, EE is fabricated by combining electrospinning and impregnation
Details
-
File Typepdf
-
Upload Time-
-
Content LanguagesEnglish
-
Upload UserAnonymous/Not logged-in
-
File Pages11 Page
-
File Size-