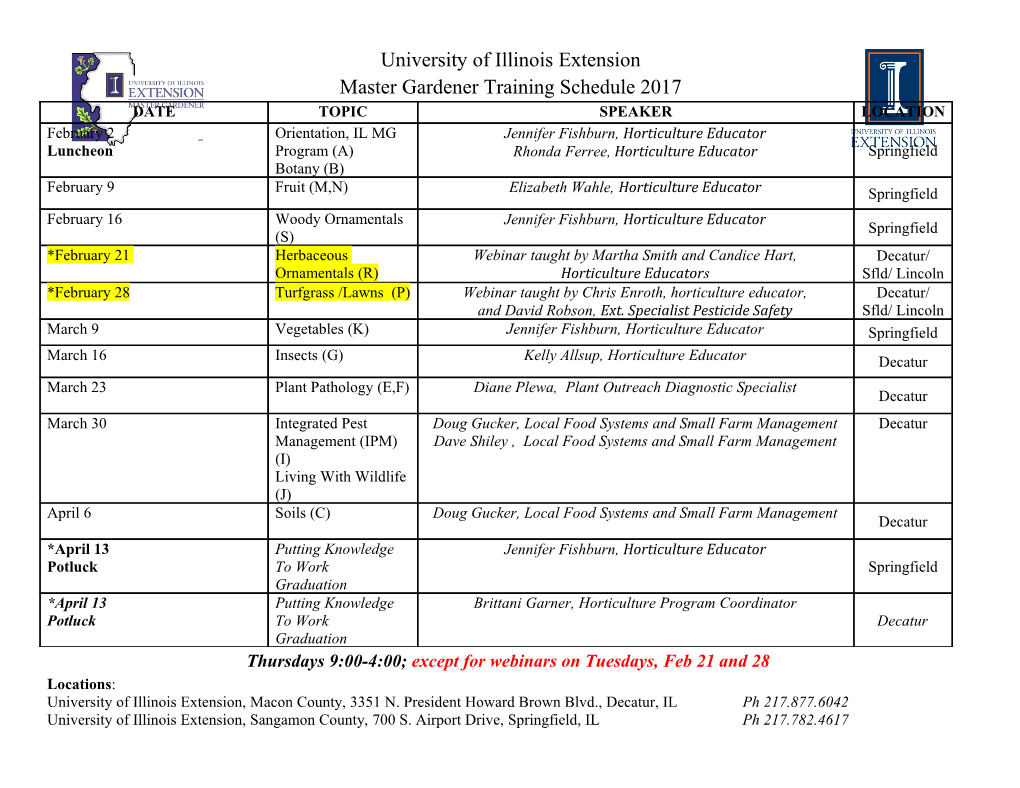
Plant mineral nutrition - AccessScience from McGraw-Hill Education http://accessscience.com/content/plant-mineral-nutrition/523500 (http://accessscience.com/) Article by: Mattson, Neil S. Department of Horticulture, Cornell University, Ithaca, New York. Last updated: 2014 DOI: https://doi.org/10.1036/1097-8542.523500 (https://doi.org/10.1036/1097-8542.523500) Content Hide Discovery of essential nutrients Absorption of nutrients by plants Links to Primary Literature Function of specific nutrients Absorption of nutrients into roots Additional Readings Nutrient deficiencies and toxicities Nutrient distribution The processes involved in plant assimilation and metabolism of all chemical elements, with the exception of carbon, hydrogen, and oxygen. The latter elements are typically excluded from the discussion of plant mineral nutrition because they are assimilated from the atmosphere and from water. Mineral nutrients are so named because they are primarily derived from the weathering of minerals of the Earth's crust, with the exception of nitrogen, which is primarily derived from atmospheric nitrogen. Taken together, these nutrient elements are critical for the processes of plant growth and hence are key to the capturing of solar energy, which is the basis of nearly all life on Earth. See also: Plant growth (/content/plant-growth /523000); Plant metabolism (/content/plant-metabolism/523400); Plant physiology (/content/plant-physiology/524500) Discovery of essential nutrients In 1699, John Woodward reported that spearmint grown in rainwater grew more poorly than plants grown in water with soil or rotting leaves. Water culture (hydroponic) techniques were subsequently employed to characterize the nature of how plants gained matter. In the nineteenth century, Justus von Liebig advanced the notion that soil per se was not required for plant growth, but that it contributed soluble inorganic constituents. Liebig further concluded that plants would die if their soil solution did not contain potassium, phosphate, and ammonium, postulating the essentiality of potassium (K), phosphorus (P), and nitrogen (N) many years before the fact was proven. Later scientists attempted to determine nutrient essentiality by growing plants in water containing all the elements that were thought to be essential, while excluding one nutrient of interest. A mineral that was found necessary for a plant to complete its life cycle was regarded as essential. The approach worked well for nutrients required in large amounts by plants, referred to as essential macronutrients (nitrogen, sulfur, phosphorus, calcium, potassium, and magnesium) [Table 1]. However, determining the essentiality of elements required in relatively small quantities, or micronutrients, required further technical developments for purifying water and salts. See also: Hydroponics (/content/hydroponics/330600) 1 of 12 6/28/17, 12:42 PM Plant mineral nutrition - AccessScience from McGraw-Hill Education http://accessscience.com/content/plant-mineral-nutrition/523500 The definition of what constitutes an essential element has been the subject of debate. In 2005, Epstein and Bloom proposed the following: “An element is essential if it fulfills either one or both of two criteria: (1) the element is a part of a molecule that is an intrinsic component of the structure or metabolism of the plant; (2) the plant can be so severely deprived of the element that it exhibits abnormalities in its growth, development, or reproduction—-that is, its ‘performance’—in comparison with plants not so deprived.” There are 14 mineral elements (excluding carbon, hydrogen, and oxygen) that are commonly accepted as being essential in higher plants. These are nitrogen, phosphorus, potassium, sulfur, calcium, magnesium, iron, manganese, zinc, copper, boron, molybdenum, chlorine, and nickel. A few additional nutrients (cobalt, selenium, silicon, and sodium) may partially substitute for one of the 14 essential elements or are beneficial to some plants under specific conditions. For example, plants have been able to complete their life cycle in hydroponic solutions with zero silicon. Yet, silicon accumulation improves disease resistance, possibly via cuticular depositions and enhancing active plant defense responses. Thus, silicon may be considered beneficial in terms of plant performance under disease pressure. Function of specific nutrients Mineral elements serve both biochemical and structural functions within a plant, with many elements serving multiple functions. Although it is difficult to succinctly classify each nutrient's role, nutrients can be placed into general categories based on function (Table 2). Class I nutrients are bound into the structure of carbon compounds, such as nucleic acids and proteins. Class II nutrients are required for energy storage and transport. Class III nutrients are linked with cell wall structure. Class IV nutrients are integral as constituents of enzymes or other molecules required for metabolism (for example, chlorophyll and ferredoxin). Class V nutrients activate enzymes or control enzyme activity. Class VI nutrients serve as major cellular osmotica. Several nutrients are placed into multiple classes. 2 of 12 6/28/17, 12:42 PM Plant mineral nutrition - AccessScience from McGraw-Hill Education http://accessscience.com/content/plant-mineral-nutrition/523500 Nutrient deficiencies and toxicities The proper concentrations of nutrients are critical for the processes involved with plant growth and metabolism. Nutrient deficiency The concentration in dry matter of a given nutrient required for adequate plant growth and metabolism varies from 0.05 to 15,000 ppm (Table 1). When a nutrient in plant tissue falls below the concentration required for optimal growth, that nutrient is said to be deficient. The metabolic processes that are directly dependent upon the nutrient and the resultant processes are slowed in plant tissue, reducing overall plant growth (Fig. 1). 3 of 12 6/28/17, 12:42 PM Plant mineral nutrition - AccessScience from McGraw-Hill Education http://accessscience.com/content/plant-mineral-nutrition/523500 Fig. 1 Plant growth in response to changes in tissue nutrient concentration. (Adapted from E. Epstein and A. J. Bloom, Mineral Nutrition of Plants: Principles and Perspectives, 2d ed., Sinauer, Sunderland, Mass., 2005) Determining the critical nutrient concentration, that is, the concentration required for near-optimal growth, in tissue for a specific plant is an area of historical and ongoing research. A tissue nutrient concentration below the critical value indicates a growth-limiting condition, which is corrected with fertilizer additions or other adjustments to the root zone. Critical nutrient concentrations vary according to plant species, developmental stage, and growth conditions. Visible symptoms resulting from a nutrient deficiency are commonly used as indicators of a nutrient disorder. For example, lack of nitrogen causes lower leaves to turn yellow. However, tissue analysis is the preferred method for interpreting a deficiency, since symptoms of many nutrient deficiencies look alike. Tissue analysis can also detect nutrient deficiencies that are not yet visibly apparent, but that could affect growth if not corrected (that is, hidden hunger). Salt and ion toxicity Mineral nutrients can be toxic to plants when they accumulate to excessive concentrations within cells. Nutrient toxicity can be direct, for example, when a specific ion interferes with plant metabolism, or indirect, as when the concentration of one or more nutrients in solution is excessive enough that it interferes with the plant's water relations. The ions of several heavy metals (cadmium, cobalt, nickel, chromium, manganese, copper, and zinc) are particularly toxic at low concentrations. Toxicity partially stems from the multivalent properties of these ions, which allow them to disrupt protein function and enzyme activity by forming cross-linkages to negatively charged sites. High concentrations of salts in the soil solution or irrigation water can impose osmotic stress, whereby the plant's water potential is depressed to such an extent that the plant cannot absorb sufficient water to grow. Plants can partially offset this effect via several mechanisms, including ion sequestration, salt excretion, and production of osmolytes, to counter the water potential difference and maintain turgor. Plants also increase production of osmoprotectants, which help maintain stable 4 of 12 6/28/17, 12:42 PM Plant mineral nutrition - AccessScience from McGraw-Hill Education http://accessscience.com/content/plant-mineral-nutrition/523500 hydration of membranes and enzymes exposed to osmotic stress. Soil salinization is estimated to reduce crop productivity on more than one-third of the world's irrigated agricultural land. See also: Plant-water relations (/content/plant-water-relations /525300); Plants of saline environments (/content/plants-of-saline-environments/525600) Absorption of nutrients by plants In order to utilize mineral nutrients for metabolism, plants must be able to absorb them and distribute them to organs within the plant. One challenge faced by plants is extracting nutrients in the correct proportions required for energy, metabolism, and growth. In most cases, nutrients are present in the soil solution in concentrations that are vastly different from the concentrations required within the plant. Plants can absorb nutrients to much higher concentrations than those in the surrounding growth substrate. For example,
Details
-
File Typepdf
-
Upload Time-
-
Content LanguagesEnglish
-
Upload UserAnonymous/Not logged-in
-
File Pages11 Page
-
File Size-