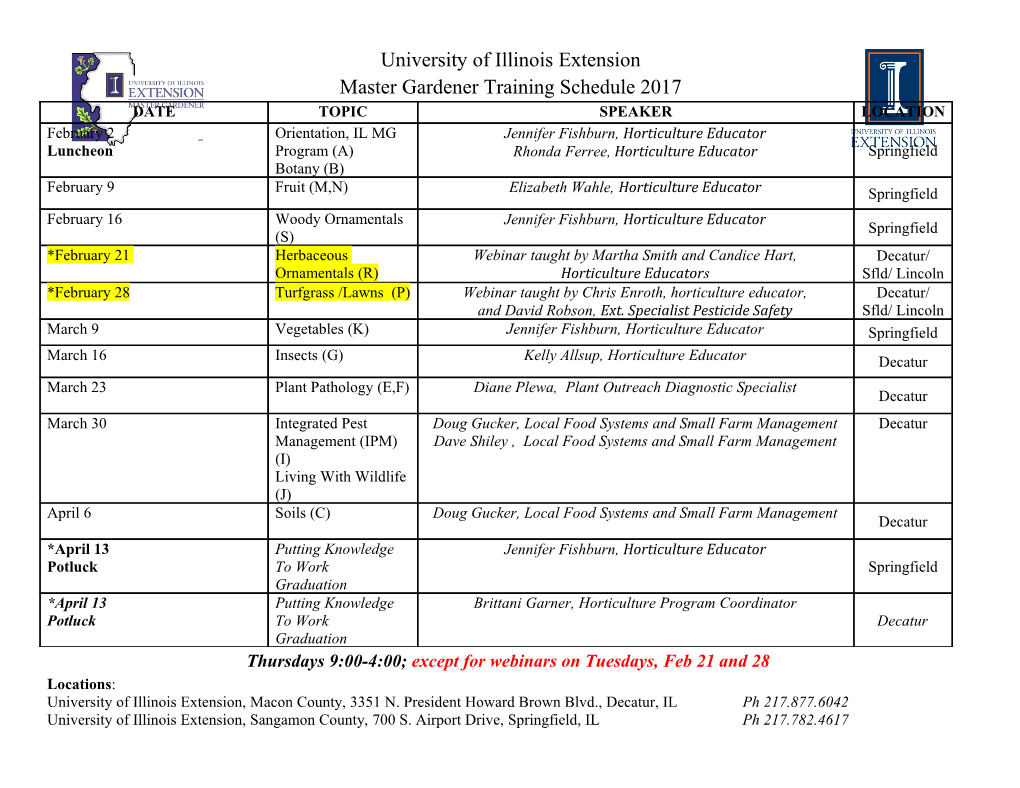
STUDIES ON DEEP ELECTRONIC LEVELS IN SILICON AND ALUMINIUM GALLIUM ARSENIDE ALLOYS Håkan Pettersson DEPARTMENT OF SOLID STATE PHYSICS LUND UNIVERSITY SWEDEN 1993 STUDIES ON DEEP ELECTRONIC LEVELS IN SILICON AND ALUMINIUM GALLIUM ARSENIDE ALLOYS av Håkan Pettersson civ. ing., Mim AKADEMISK AVHANDLING SOM FÖR AVLÄGGANDE AV TEKNISK DOKTORSEXAMEN VID TEKNISKA FAKULTETEN VID UNIVERSrTETET I LUND KOMMER ATT OFFENTUGEN FÖRSVARAS I FYSISKA INSTrrtrnONENS FÖRELÄSNINGSSAL B FREDAGEN DEN 14 MAJ 1993, KL. 10.15. \i t1*. p LUND UNIVERSITY DOCTORAL DISSERTATION Department of Solid State Physics Date of Box 118 S-221 00 Lund Sweden LUFTD2/TFFF-QQ38/1-1SS A«tfcM(*) Håkan Pettersson Studies on Deep Electronic Levels in Silicon and Aluminium Gallium Arsenide Alloys Abstract " " This thesis reports on investigations of the electrical and optical properties of deep impurity centers, related to the transition metals (TMs) Ti. ftft>- W. V and Ni. in silicon. Emission rates, capture cross sections and photoionization cross sections for these impurities were determined by means of various Junction Space Charge Techniques (ISCTs). such as Deep Level Transient Spectroscopy (DLTS). dark capacitance transient and photocapacitance transient techniques. Changes in Gibb's free energy as a function of temperature were calculated for all levels. From mis temperature dependence, the changes in enthalpy and entropy involved in the electron and hole transitions were deduced. The influence of high electric fields on the electronic levels in chalcogen-doped silicon were investigated using the dark capacitance transient technique. The enhancement of the electron emission from the deep centers indicates a more complex field enhancement model than the expected a Poole-Frenkel effect for coulombk potentials. The possibility to determine charge states of defects using the Poole-Frenkel effect, as often suggested, is therefore questioned. 3 The observation of a persistent decrease of the dark conductivity due to illumination in simplified AlGaAs/GaAs High Electron Mobility Transistors (HEMTs) over the temperature range 170K<T<300K is reported. A model for this peculiar behavior, based on the recombination of electrons in the two-dimensional electron gas (2DEG) located at the AlGaAs/GaAs interface with holes generated by a 2? two-step excitation process via the deep EL2 center in the GaAs epilayer, is put forward. Keywonbpeep impurity center, TM, silicon, emission rate, capture cross section, photo- ionization cross section, JSCT, DLTS, dark capacitance, photocapacitance, Gibb's free energy, enthalpy, entropy, Poole-Frenkel effect, AlGaAs/GaAs, HEMT, 2DEG, EL2. Cturification lystcm and/or index terms (if aay) Supplementary bibliographical information Uofuafe Enallsh ISSN and key title ISBN Recipknl's notes Number of pages Price 155 Security cbuffieatiofl DUributkm by (name and addres) Department of Solid State Physics, Lund University, Box 118, S-221-00 Lund, Sweden I, the undenJgned, being the copyright owner of the abstract of the above-mentioned disjertatioa, bereby grant t« ail reference fourco permiiMOfl to publish and dJamninate the abstract of the above-mentioned diaierUtion. Date April 15, 1993 v STUDIES ON DEEP ELECTRONIC LEVELS IN SILICON AND ALUMINIUM GALLIUM ARSENIDE ALLOYS ty Hakan Pettersson Lund 1993 v Department of Solid State Physics University of Lund Box 118 S-221G0Lund Sweden © Håkan Pettersson 1993 ISBN 91-628-0883-4 KF-SIGM A LIH, Lund 1993 Printed in Sweden .••/• Studies on Deep Electronic Levels in Silicon and Aluminium Gallium Arsenide Alloys by Håkan Pettersson Department of Solid State Physics, University of Lund Box 118, S-221 00 Lund, Sweden This thesis reports on investigations of the influence of high electric fields on y the electronic levels in chalcogen-doped silicon (paper I), electrical and optical properties of some transition metal related defects in silicon (papers II-V), and a study of a light induced anomaly in modulation-doped AIGaAs/GaAs heterostructures (paper VI and VII). The thesis consists of the following seven papers: I Electric-field-enhanced electron emission from isolated sulfur and selenium donors in silicon (Phys>. Rev. B 42,1381 (1990). II Electrical and optical properties of titanium-related centers in silicon (Phys. Rev. B 43,9171 (1991). IH Electrical and optical properties of molybdenum and tungsten related defects in silicon (Semicond. Sci. Technol. 6, 237 (1991). • IV Electrical and optical properties of vanadium-related centers in silicon (Phys. Rev. B 44,12809 (1991). ! V Electrical and Optical Characterization of Niobium-related Centers in Silicon i (accepted for publication in Semicond. Sci. Technol.) VI Observation of a Persistent Negative Photoconductivity Effect in AIGaAs/GaAs Modulation-Doped Structures (Phys. Rev. Lett. 67, 3010 (1991). VII Persistent Decrease of Dark Conductivity due to Illumination in AIGaAs/GaAs Modulation-Doped Heterostructures (submitted to J. Appl. Phys.). Co-authors are H. G. Grimmeiss (I-VII), L. Tilly (II-V), K. Schmalz (II-V), K. Tittelbach (II-IV), H. Kerkow (II-V), A. Powell (VI, VII), C. C. Button (VI, VD), J. S. Roberts (VI, VE) and P. I. Rockett (VI, VII). A contribution to the MRS Spring Meeting, San Fransisco (1992), is left out of tias thesis because the topic is covered in papers II-IV. VIII Electrical and optical properties of titanium, vanadium, molybdenum, and tungsten related defects in silicon (invited). /• t , tv* The following eight chapters give an introduction to the experimental work presented in this thesis. 1. INTRODUCTION 4 2. THE MATERIALS 9 2.1 Silicon and gallium arsenide crystals - Physical aspects 9 2.2 Crystal growth 13 2.3 Doping of semiconductors 15 3. THE ELECTRONIC STRUCTURE OF POINT DEFECTS IN SEMICONDUCTORS 16 3.1 The energy spectrum of shallow impurities 16 3.2 Limitations of EMT-Deep levels 21 3.3 Transition metals in silicon 22 4. ELECTRICAL PROPERTIES OF POINT DEFECTS IN SEMICONDUCTORS 27 4.1 Shockley-Read statistics 27 4.2 Physical interpretation of the electronical parameters. 30 4.3 The Poole-Frenkel Effect 34 5. SAMPLE STRUCTURES 37 6.1 The Diode 37 40 6.2 The High Electron Mobility Transistor (HEMT) 43 6. MEASUREMENT TECHNIQUES 52 7. SUMMARY OF PAPERS 57 8. LIST OF REFERENCES 62 ACKNOWLEDGEMENTS 63 APPENDED PAPERS %"* 1. INTRODUCTION Solidified condensed matter can be divided into three groups; crystalline, polycrystalline and amorphous materials. A crystal is constructed by the infinite repetition in space of identical building blocks. This building block, in the case of copper or silver, may be one single atom, but it may also be as many as 10 000 atoms for a protein crystal. Polycrystalline materials, like silicon dioxide, consist of small crystals randomly oriented in space. Finally, in amorphous materials, like glass, all the atoms are randomly oriented in space. Crystalline materials can be further divided into two sub-groups, metals and non-metals. Metals are characterized by high electrical conductivity which means that a large number of conduction electrons must be free to move through the crystal. It is the weakly bound valence electrons of the free atom which become the conduction electrons in the metal. Also, it is this "sea" of free electrons which acts as a glue and binds together the crystal. In a non-metal there are almost no free electrons and therefore it has a poor electrical conductivity. In these crystals the valence electrons are either bound in covalent bonds (valence crystals) or in atomic closed shells (ionic crystals). These crystals will be discussed more in detail in the next chapter. The energy required to break one of these bonds to release an electron varies between different crystals but in general the ionic crystals are more strongly bound. Most of the important electrical and optical properties of crystals can be easily understood if the so called band model of the crystal is used. As free atoms are brought closely together to form a crystal, the discrete energy levels of the single atoms start to interact with each other, which leads to the formation of energy bands. This is schematically depicted in Fig. 1.1. First excited .^^•M^^^H^H^H banConductiod n state ^^L^HHHHB 1 Bandgap Ground state <••••••••••••••••••••I ^^^^^^^^^^^^^^B Valence ^•^^^^•^^^H band Atomic distance Free atom Solid crystal Fig.1.1: Schematic description of the formation of energy bands in crystals. The most important bands are the valence band and the conduction band. The bottom of the conduction band is separated from the top of the valence band by the so called bandgap. The bandgap is an energetically forbidden area for electrons in the sense that no electron can have an energy within the bandgap (this statement will be somewhat modified later). The valence electrons from all the atoms of the crystal fill the bands to an extent determined by the number of valence electrons that each atom possesses. Electrons within a band can be accelerated by an external electric field, which is a prerequisite for a high conductivity, unless the band is completely filled. The valence and ionic crystals have filled valence bands (and empty conduction bands), and therefore a poor conductivity. However, if an electron in the valence band is provided with enough energy to overcome the bandgap it may enter the conduction band where it can be accelerated. In this case the emitted electron leaves behind a so called hole in the valence band. This hole, which simply is an absent electron, can be thought of as a positively charged particle which can gain energy analogously to the electron in the conduction band. The emission of an electron to the conduction band is in the "bond model" equivalent to breaking one of the crystal bonds to release an electron and leave a hole behind. One can easily visualize the motion or a hole in the bond model as the motion of an electron from a nearby bond to the site of the missing electron, i.
Details
-
File Typepdf
-
Upload Time-
-
Content LanguagesEnglish
-
Upload UserAnonymous/Not logged-in
-
File Pages156 Page
-
File Size-