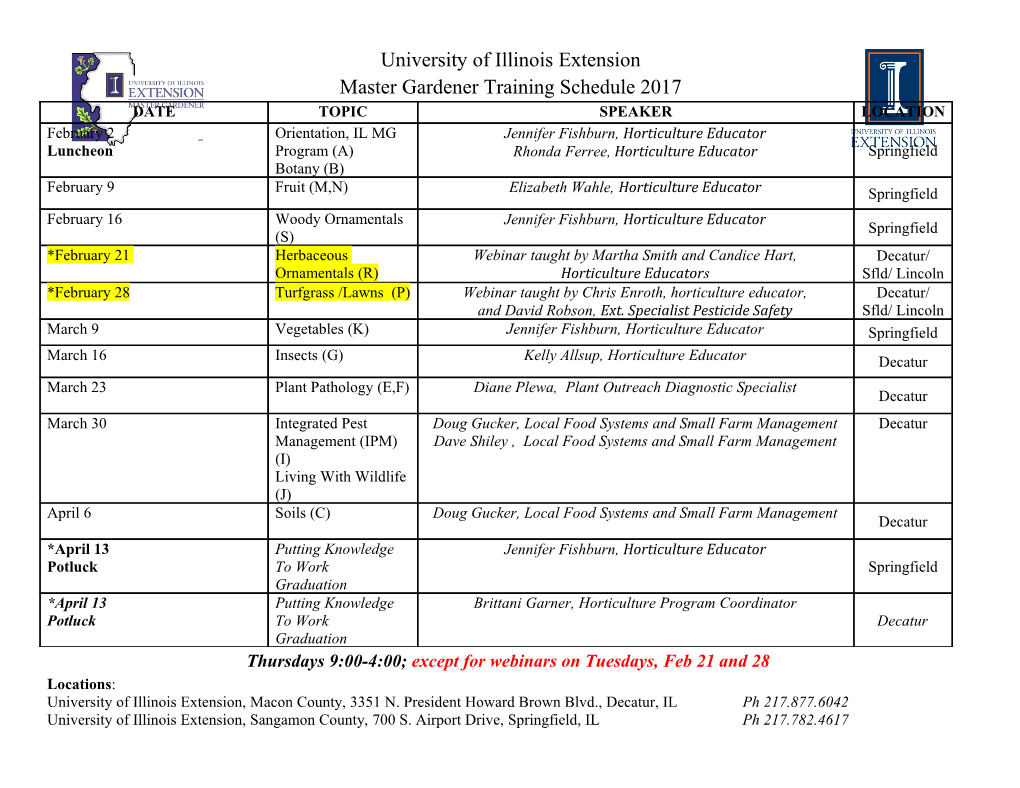
Workshop Lecture Journal of Inorganic Biochemistry 96 (2003) 3 Structural Genomics Antonio Rosato, Magnetic Resonance Center, University of Florence, Italy To realize the true value of the wealth of data provided by genome sequencing data, it is necessary to relate them to the functional properties of the proteins they encode. Since the biological function of a protein is determined by its 3D structure, the systematic determination of proteins’ structures on a genome-wide scale is a crucial step in any (post-)genomic effort, which may (or may not) provide initial hints on the function. This is what is commonly referred to as ‘Structural Genomics’ (or Structural Proteomics). Because of the huge number of systems into question, all the complex steps necessary for structure determination must be optimized, streamlined and, possibly, robotized in order to shrink the time needed to solve each protein structure. This approach is dubbed ‘high-throughput’ (HTP) and is an intrinsic feature of Structural Genomics. What can be the relationship between Biological Inorganic Chemistry and Structural Genomics? A major challenge is that to reconcile the concept of HTP with the care that metalloproteins most often require because of their metal cofactors. The identifi cation of metalloproteins is even not explicitly taken into account in purely Structural Genomics projects, nor is any methodology particularly developed for them. To create true correlations between Biological Inorganic Chemistry and Structural Genomics it is necessary to develop new computational tools (e.g. to identify metalloproteins in databanks, or to correctly model their structures), as well as new methodological approaches to HTP metalloprotein expression/purifi cation and structural characterization. Of course, focus on the metal site, and its interplay with the protein moiety, is of fundamental importance. On the longer term, these developments will be crucial to unravel the complex mechanisms underlying the interactions between metal ions and bio-molecules in living cells, even though they may fail to provide information on the metal(s) actually bound in vivo. References: Bertini and Rosato, PNAS, 100:3601-4, 2003; Banci and Rosato, Acc.Chem.Res, 36:215-221, 2003. Plenary Lectures Journal of Inorganic Biochemistry 96 (2003) 7 Scanning Probe Microscopy and the Electrochemistry of Metalloproteins and Metalloenzymes: Seeing is Believing? Allen Hill, Oxford University, United Kingdom The development of bioelectrochemistry over the past quarter of a century has concentrated on the behaviour of metalloproteins and metalloenzymes at electrode surfaces and, of course, the applications to bioanalytical chemistry. However, our understanding of such systems rests on the behaviour of such systems at, or on, electrode surfaces. The advent of methods of applying Scanning Probe Microscopy in water opened up the possibility of studying biological molecules under conditions appropriate to their natural functions and hence one could assume that they were not signifi cantly changed in their structures or functions. Though the fi rst protein examined was the zinc-containing protein, metallothionein, we have concentrated on azurin and cytochrome P450 and its partner proteins, putidaredoxin (Pd)and putidaredoxin reductase (PdR). In particular, we have sought conditions that allow the investigation of structures of the complexes formed between cytochrome P450 and Pd and PdR. With all these expermiments, it was important to show that the proteins were still active when adsorbed on the electrode surface and, as expected, it was usually important to modify the surface with a promoter (or, more recently, a facilitator.) We are now attempting to study the electrochemistry of the proteins under these conditions and to move the proteins into organised arrays in preparation for the use of similar systems as truly molecular sensors. The investigation of the ubiquitous glucose sensor by Scanning Tunneling Microscopy under conditions closely related to those found in devices proved possible. Some New Aspects of Molecular Engineering of Heme Proteins to Manipulate Their Functions: Ligand Binding, Catalysis and Interprotein Recognition Isao Morishima, Kyoto University, Japan We have been concerned with rational redesign of the structures of heme proteins in order to better understand the chemical diversity of natural heme proteins and to generate novel heme proteins of potential utility. Detailed structural analysis of proteins have revealed that proteins structures are constructed by ‘modules’, which are compact structural units and correspond to exons on gene structure. In addition to the conventional point mutation, the approach we have developed is the ‘module’ substitution on the protein structure as the effective rational design step, and is, if necessary, followed by the second step, the point or random mutations for the fi ne-tuning. By inserting or substituting the specifi c module playing an important role in a desired function, we can expect that the starting point might jump to the goal. We have studied molecular engineering of heme proteins to better understand P450cam catalyzed oxygenation reactions with particular focus on putidaredoxin (electrondonor)-induced conformational changes of P450cam, to convert horseradish peroxidase to catalase and to position the oxidizing equivalents in peroxidase to change the reactivity. We have designed and created the calcium binding site in myoglobin in which the calcium binding module of troponin c is implanted into myoglobin and oxygen binding affi nity is modulated by calcium binding. We have also extensively studied the module-substituted chimeric globins to change the protein-protein recognition characteristics. For example, the module-substituted b subunit of hemoglobin or myoblobin having the module M4 from the a-subunit assembled with the b-subunit, indicating that the module M4 plays a crucial role in the specifi c subunit association in globins. Some examples of the chimeric myoglobin exhibiting association properties with other proteins will be presented. Some of the chimeric substitutions among different proteins with no homology have resulted in quite unstable proteins. We have developped the irrational design by the fi ne-tuning using the directed evolution, which allows us to construct novel and stable chimeric proteins between the different kinds of proteins. 8 Journal of Inorganic Biochemistry 96 (2003) Chromium in Biology – Toxicology and Nutritional Aspects Peter A Lay, University of Sydney, Australia Chromium is probably the most controversial of the transition metal ions in terms of its toxicity and nutritional value.1,2 Cr(VI) compounds constitute one of the fi rst classes of chemicals to be implicated as human carcinogens, whereas Cr(III) is considered as one of the least-toxic transition metals and its use as a dietary supplement in nutrition and diabetes treatment is both very controversial and the basis of a multi-million dollar industry.1 Apart from occupational health issues, the controversy over industrial uses of Cr(VI) has also escalated as a result of recent massive lawsuits in the USA involving environmental pollution that has adversely affected local populations.2 The literature on both the mechanisms of chromium-induced cancers and the use of Cr(III) as dietary supplements is full of diverse opinions and confl icting reports.1 The divergent opinions can, in part, be attributed to the complexity of Cr redox and substitution chemistry in vitro and in vivo and, in part, to the diverse scientifi c backgrounds from which different research groups tackle the problems posed by Cr bioinorganic chemistry. This talk will concentrate on new developments in Cr carcinogenicity and nutritional supplements and on the need to investigate such complex bioinorganic questions using a multi-disciplinary approach. This is required to provide real insights into understanding the bioinorganic chemistry of Cr (and other metals) in biology and medicine. Acknowledgments: We are grateful for fi nancial support from the Australian Research Council, National Health and Medical Research Council, the Australian Synchrotron Research Program and the Access to Major Facilities Program. We are also grateful for beamtime at the Advanced Photon Source and the Stanford Synchrotron Research Laboratory. 1 Codd, R.; Dillon, C. T.; Levina, A.; Lay, P. A. Coord. Chem. Rev. 2001, 216-217, 537-582. 2 Levina, A.; Codd, R.; Dillon, C. T.; Lay, P. A. Prog. Inorg. Chem. 2003, 51, 145-250. Radicals and Metal Centers as Spectroscopic Probes for Structure and Function of Photosynthetic Reaction Centers Wolfgang Lubitz, Max-Planck-Institut für Strahlenchemie, Mullheim, Germany The reaction centers (RCs) of photosynthetic organisms are highly complex multisubunit integral membrane proteins with several cofactors that are actively involved in the conversion of sun light into chemical energy. In oxygenic photosynthesis two photosystems (PS I and PS II) exist, which have recently been crystallized with 2.5 ≈ and 3.8 ≈ resolution, respectively [1,2]. The major pigment chlorophyll a is involved in light harvesting and excitation transfer in the antenna, and acts both as electron donor and intermediate electron acceptor in the charge separation process. The stable electron acceptors are quinone molecules coupled to a non-heme iron in PS II and three iron-sulfur clusters in PS I. Light-induced charge separation in PS II leads to oxidation of water and the release of protons and molecular
Details
-
File Typepdf
-
Upload Time-
-
Content LanguagesEnglish
-
Upload UserAnonymous/Not logged-in
-
File Pages77 Page
-
File Size-