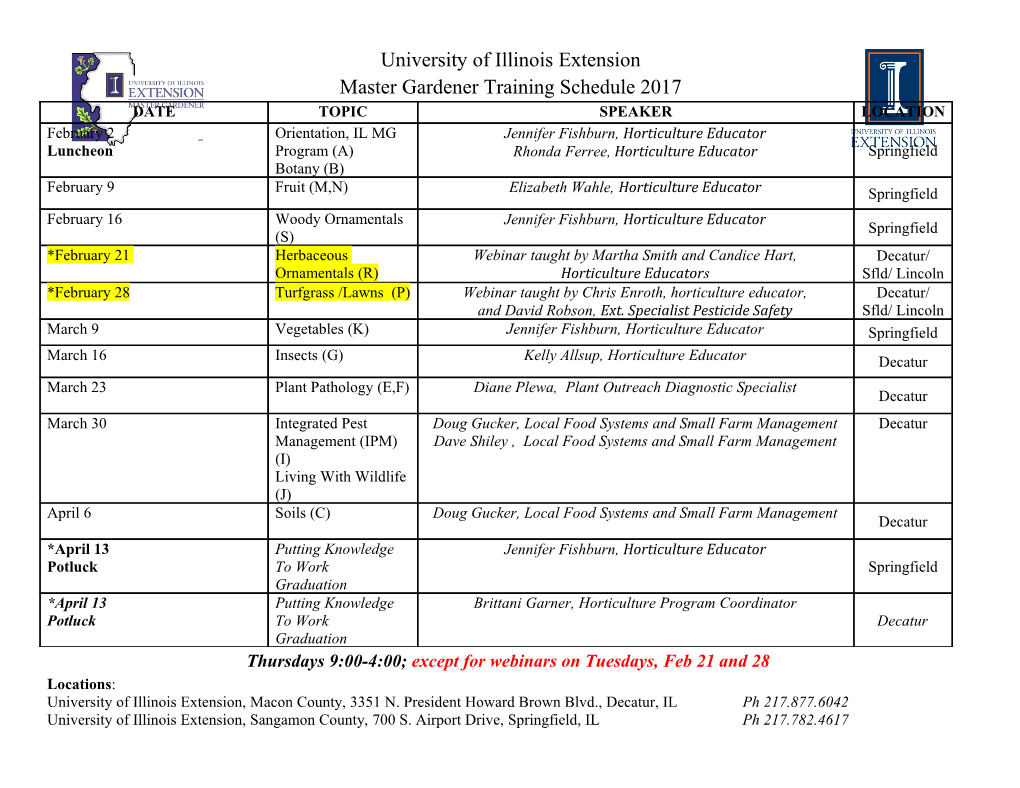
Confidential manuscript submitted to Geophysical Research Letters 1 The dominant role of the East Siberian Sea in driving the oceanic flow through the Bering 2 Strait – conclusions from GRACE ocean mass satellite data and in situ mooring 3 observations between 2002 and 2016. 4 5 Cecilia Peralta-Ferriz1 and Rebecca A. Woodgate1 6 1Polar Science Center, Applied Physics Laboratory, University of Washington. 7 Corresponding author: Cecilia Peralta-Ferriz ([email protected]) 8 Key Points: 9 Ocean mass data show monthly variability in the Bering Strait throughflow to be primarily 10 driven from the Arctic, not the Pacific. 11 ~2/3rds of summer flow variability is driven by oceanic sea-level changes in the East Siberian 12 Sea, associated with westward Arctic winds. 13 In winter, northward Bering Strait winds and North Pacific cyclonic winds (raising sea-level over 14 the Bering Sea Shelf) also contribute. 15 16 Abstract 17 It is typically stated that the Pacific-to-Arctic oceanic flow through the Bering Strait (important for 18 Arctic heat, freshwater, and nutrient budgets) is driven by local wind and a (poorly defined) far-field 19 “pressure-head” forcing, related to sea-surface-height differences between the Pacific and the Arctic. 20 Using monthly, Arctic-wide, ocean bottom pressure satellite data and in situ mooring data from the 21 Bering Strait from 2002-2016, we discover the spatial structure of this pressure-head forcing, finding 22 that the Bering Strait throughflow variability is dominantly driven from the Arctic, specifically by sea- 23 level change in the East Siberian Sea (ESS), in turn related to westward winds along the Arctic coasts. In 24 the (comparatively calm) summer, this explains ~2/3rds of the Bering Strait variability. In winter, local 25 wind variability dominates the total flow, but the pressure-head term, while still correlated with the ESS- 26 dominated sea-level pattern, is now more strongly related to Bering Sea shelf sea-level variability. 1 Confidential manuscript submitted to Geophysical Research Letters 27 1 The importance of the Bering Strait throughflow and its driving mechanisms 28 The (generally northward) flow through the Bering Strait is the only oceanic Pacific input to the Arctic. 29 Although comparatively small in volume (~1Sv, 1Sv=1x106m3/s) [Roach et al., 1995], the throughflow 30 dominates the water properties of the Chukchi Sea [Woodgate et al., 2005b] and the upper (<150m) 31 layer of the western Arctic Ocean [Woodgate, 2013]; is a key source of nutrients for the Arctic and 32 beyond [Torres-Valdés et al., 2013; Walsh et al., 1997]; provides about 1/3rd of the freshwater entering 33 the Arctic [Aagaard and Carmack, 1989; Serreze et al., 2006] and enough oceanic heat to influence 34 western Arctic sea-ice retreat [Woodgate et al., 2010]; and contributes to the stability of the Atlantic 35 meridional overturning circulation and world climate [De Boer and Nof, 2004b; Hu et al., 2007]. 36 While short-term (timescales < weeks) variability in the throughflow has long been attributed to the 37 local wind variability [see Woodgate et al., 2005b, for discussion], at times of negligible wind in the 38 strait, the flow is still northward, and this is generally conceptually attributed to a far-field, sea-surface 39 height (SSH) “pressure-head” difference between the Pacific and the Arctic [Coachman and Aagaard, 40 1966; Stigebrandt, 1984]. However, the origins of this pressure-head – variously hypothesized to be 41 steric [Aagaard et al., 2006; Wijffels et al., 1992] or set up by global wind patterns [De Boer and Nof, 42 2004a; b] – are still obscure. And given the complex SSH fields both north and south of the strait [e.g., 43 Danielson et al., 2014], it is far from clear between what points such a pressure-head should be 44 measured. Prior SSH studies have evaded this question either by considering only SSH gradients across 45 the strait proper (e.g., Cherniawsky et al. [2005], who suggest the across-strait gradient is in general 46 geostrophic balance with the flow, a result consistent with in situ current and pressure gauge 47 measurements [Woodgate, pers. comm.]), or SSH averages across the Bering Sea, Chukchi Sea or 48 Beaufort Gyre [e.g., Danielson et al., 2014]. The latter study, the most extensive to date, concludes – as 49 has been the focus of most prior work – that changes in the pressure-head forcing are primarily driven 50 from the south, i.e., the Bering Sea and/or North Pacific. 51 We bring now a new data set to this discussion, viz. a global, satellite-based measure of the Earth’s 52 time-varying gravity field, as obtained by the Gravity Recovery and Climate Experiment (GRACE) from 53 2002 to 2016 [Tapley et al., 2004]. In ocean regions, this yields temporal (typically 1 month averages) 54 and spatial (~300km resolution) information about variability in ocean bottom pressure, a key 55 component for understanding the pressure-head forcing of the Bering Strait throughflow. 2 Confidential manuscript submitted to Geophysical Research Letters 56 In this paper, we combine observations of GRACE ocean bottom pressure with in situ observations of 57 the Bering Strait velocity (taken from the year-round moorings deployed in the strait since 1990, see 58 Woodgate et al. [2015], for overview) and atmospheric reanalysis data to consider drivers of monthly to 59 seasonal variability of the Bering Strait throughflow over a 14-year period, 2002-2016. 60 2 Data Sets: GRACE Ocean Bottom Pressure, atmospheric reanalysis data, and in situ mooring 61 data 62 This study uses three independent data sets: 63 2.1 GRACE Ocean Bottom Pressure anomalies (OBP) 64 We use monthly composites (March 2002 – June 2016) of GRACE Ocean Bottom Pressure anomalies 65 (OBP) from the Center for Space Research (CSR), specifically the Release 5 “mass concentration 66 blocks” (mascons) solutions (http://www2.csr.utexas.edu/grace/RL05_mascons.html). While available 67 on a global 0.5° grid, the native resolution of mascons is greater, ~120km, and the effective resolution is 68 possibly larger (~300km) [Save et al., 2016]. We test the results of our analysis using also the GRACE 69 CSR spherical-harmonic product [Chambers and Bonin, 2012], and the GRACE Jet Propulsion 70 Laboratory mascon product [Watkins et al., 2015]. Despite the different processing of these products, 71 they give very consistent results in our analysis. 72 Units of OBP are equivalent water thickness (in cm, assuming a water density of 1028kg/m ). Estimated 73 uncertainties for mascons are <2cm [Save et al., 2016]. To avoid issues of converging lines of longitude, 74 we linearly interpolate the final product onto a ~50km resolution regular grid centered on the North 75 Pole. The GRACE data are provided as anomalies from the January 2005 to December 2010 mean, this 76 being the largest gap-free period of GRACE measurements. For our analysis, we also detrend the time- 77 series at each point. 78 2.2 Atmospheric reanalysis data 79 As per prior work [e.g., Woodgate et al., 2006], we use NCEP (National Center for Environmental 80 Prediction) atmospheric reanalysis products [Kalnay et al., 1996], 81 (http://www.esrl.noaa.gov/psd/data/gridded/data.ncep.reanalysis.html) at 6-hourly temporal and 2.5deg 82 horizontal resolution. We compute (and interpolate onto our 50km regular grid) monthly-averaged sea- 83 level pressure (SLP), zonal (positive eastward, Uwinds) and meridional (positive northward, Vwinds) 3 Confidential manuscript submitted to Geophysical Research Letters 84 winds at 925-mb height, from 2002 to 2016, and from 30°N to the North Pole. While other wind 85 products could be used in the strait, in other Bering Strait work comparable results are found with 86 various wind-products in the region [Woodgate, pers. comm.], and in the high Arctic, Lindsay et al. 87 [2014] find NCEP wind-speed data are well correlated (r~0.8) with local observations. 88 2.3 Bering Strait Mooring data 89 We use monthly averages of northward water velocity in the Bering Strait, obtained from a year-round 90 in situ “climate” mooring in the central strait (site A3) [for overview see Woodgate et al., 2015]. Away 91 from the seasonal boundary currents, the velocity field in the strait is strongly barotropic and highly 92 homogeneous (velocity correlations ~0.9), and thus A3 northward velocity is shown to be a good proxy 93 of the total transport through the strait [Woodgate et al., 2015; Woodgate et al., 2012]. 94 We also use a mooring-based estimate of the part of the flow driven by the far-field pressure-head 95 (denoted here by PHterm), calculated as the residual after removing from the northward in situ mooring 96 velocity the variability correlated with the local 6-hourly NCEP wind forcing (best correlations found 97 with wind heading 330°N), as per Woodgate et al. [2005b], Woodgate et al. [2006]. The variance of the 98 monthly-averaged PHterm is roughly ~50%, ~75% and ~45% of the variance of the total flow for the 99 year-round, summer and winter seasons, respectively. 100 Hourly Bering Strait mooring data are available at the National Centers for Environmental Information, 101 http://www.nodc.noaa.gov, and monthly averages of both data and the PHterm are available at the 102 Bering Strait project website, http://psc.apl.washington.edu/BeringStrait.html. 103 Throughout the paper, for correlations we consider as noteworthy only those which are significant at the 104 95% confidence level or above. 105 3 Methodology 106 Our goal is to ascertain to what extent variability in the Bering Strait throughflow is related to patterns 107 and variability of OBP and, where possible, identify associated atmospheric forcings.
Details
-
File Typepdf
-
Upload Time-
-
Content LanguagesEnglish
-
Upload UserAnonymous/Not logged-in
-
File Pages21 Page
-
File Size-