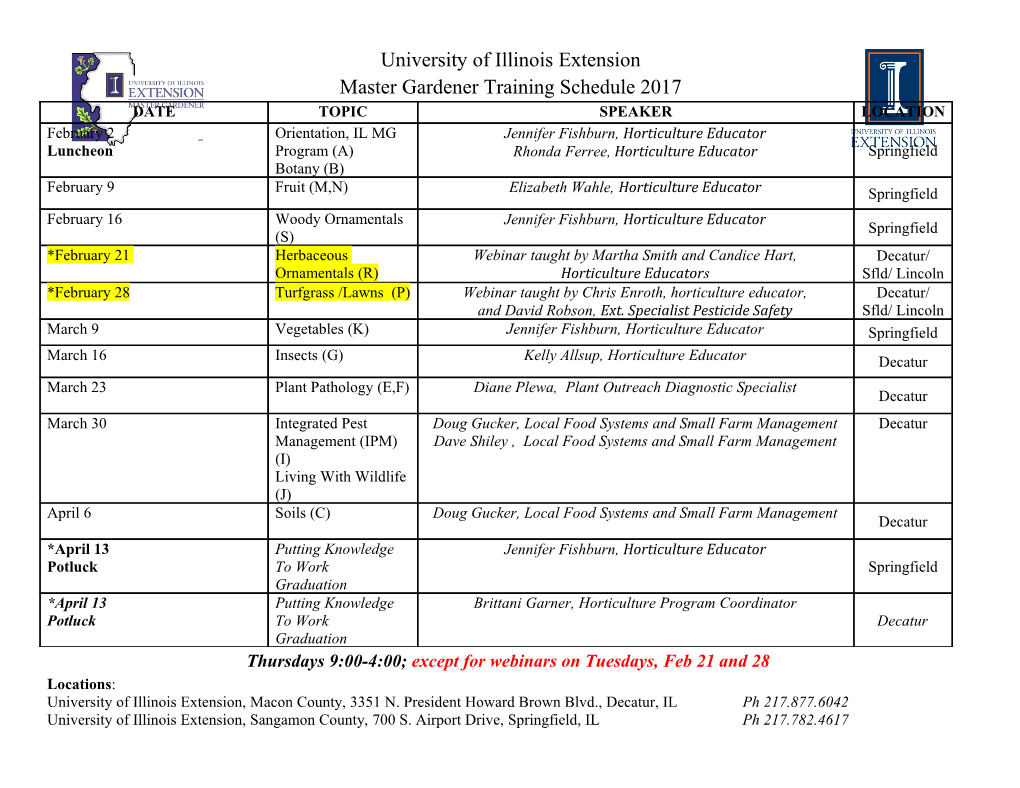
Elementary Reaction Models for CO Electrochemical Oxidation on an Ni/YSZ Patterned Anode The MIT Faculty has made this article openly available. Please share how this access benefits you. Your story matters. Citation Shi, Yixiang, et al. “Elementary Reaction Models for CO Electrochemical Oxidation on an Ni/YSZ Patterned Anode.” ASME 2010 8th International Fuel Cell Science, Engineering and Technology Conference: Volume 2, 14-16 June, 2010, Brooklyn, New York, ASME, 2010, pp. 159–66. © 2010 by ASME As Published http://dx.doi.org/10.1115/FuelCell2010-33205 Publisher ASME International Version Final published version Citable link http://hdl.handle.net/1721.1/119260 Terms of Use Article is made available in accordance with the publisher's policy and may be subject to US copyright law. Please refer to the publisher's site for terms of use. Proceedings of the ASME 2010 Eighth International Fuel Cell Science, Engineering and Technology Conference FuelCell2010 June 14-16, 2010, Brooklyn, New York, USA FuelCell2010-33205 ELEMENTARY REACTION MODELS FOR CO ELECTROCHEMICAL OXIDATION ON AN NI/YSZ PATTERNED ANODE Yixiang Shi1,2 Won Yong Lee Ahmed F. Ghoniem 1 Department of Mechanical Engineering Department of Mechanical Engineering Department of Mechanical Engineering Massachusetts Institute of Technology, Massachusetts Institute of Technology, Massachusetts Institute of Technology, Cambridge, MA, USA, 02139 Cambridge, MA, USA, 02139 Cambridge, MA, USA, 02139 2 Department of Thermal Engineering, Tsinghua University, Beijing, China, 100084 measurements for CO electrochemical oxidation on ABSTRACT nickel/yttria-stabilized zirconia (Ni/YSZ) patterned anode, and examine Analysis of recent experimental impedance spectra and possible limiting steps in CO oxidation kinetics. We consider four polarization curves of nickel/yttria-stabilized zirconia (Ni/YSZ) possible reaction mechanisms for CO electrochemical oxidation at Ni/YSZ anode, as shown in Table 1. Mechanism I is taken from Etsell patterned anode of a solid oxide fuel cell (SOFC) are used to determine 12 the limiting steps in CO electrochemical oxidation kinetics. A and Elengas , and involves gaseous species or species adsorbed on the surface of the electrode and/or the electrolyte. In mechanism II, the comprehensive 1D model is proposed for the prediction of the 2- steady-state polarization curve of a patterned anode SOFC. The model oxidation of adsorbed CO by O in the electrolyte is proposed. incorporates gas species adsorption/desorption with surface diffusion Mechanism Is and IIs are constructed by adding the surface diffusion and one of two possible charge transfer reaction steps: O charge steps to Mechanism I and II, respectively. These kinetic mechanisms transfer reaction [O2-(YSZ)+(Ni)↔(YSZ)+O(Ni)+2e-], or CO charge are illustrated in Fig. 1, in which the geometry is similar to that used in 2- - the experimental setup of the patterned Ni anode button cell on a YSZ transfer reaction [O (YSZ)+CO(Ni)↔(YSZ)+CO2(Ni)+2e ]. We show single crystal with LSM/YSZ porous cathode investigated by that the mechanism incorporating charge transfer between adsorbed CO 11 and oxygen vacancy is able to better predict the experimental data. We Habibzadeh et al. estimate some of the model parameters, such as the exchange current density and charge transfer coefficient by fitting the simulation of the polarization curves to the experimental data. Key Words: solid oxide fuel cells, carbon monoxide oxidation, patterned electrode, charge transfer, surface diffusion 1. Introduction Solid oxide fuel cells (SOFCs) are known for their fuel flexibility and tolerance to carbon monoxide, CO. Thus, syngas can be used in an SOFC directly in generating electricity. Syngas, a mixture of carbon monoxide, CO, and hydrogen, H2, is derived from steam reforming of coal, biomass, methane, or other hydrocarbons.1 In order to optimize the anode performance, it is important to construct Figure 1. Patterned Ni patterned anode geometry and elementary comprehensive electrochemical oxidation models H2 and CO near the three-phase boundary (TPB) of the SOFC, based on sound reaction pathways experimental data and kinetic mechanisms. Compared with the systematic experimental and theoretical studies of H2 electrochemical We propose an 1D comprehensive electrochemical oxidation oxidation 2,3,4,5,6,7,8,9,10, few studies have been performed for the CO model for the prediction of the steady-state polarization curve of a electrochemical oxidation mechanism. patterned anode SOFC. The model utilizes one of the CO Different reaction mechanisms have been proposed for CO electrochemical oxidation mechanism described in Table 1 and electrochemical oxidation, depending on the experimental conditions incorporates either of the two charge transfer steps shown in Table 1, and the cell material. To reduce the effects of gas-phase mass transport that is: the O charge transfer or the CO charge transfer, as well as on the impedance, and eliminate some of the ambiguities in the analysis surface diffusion processes. Some of the model parameters are given of electrochemical oxidation rates, Habibzadeh 11 studied the CO and others are adjusted to match experimental polarization curves. We electrochemical characteristics on a Ni patterned anode. In this paper, use the experimental data to determine the electrochemical mechanism we use their experimental impedance spectra and polarization curve most likely to predict the measurements accurately. 1 Copyright © 2010 by ASME Downloaded From: https://proceedings.asmedigitalcollection.asme.org on 11/20/2018 Terms of Use: http://www.asme.org/about-asme/terms-of-use occupied by a single CO molecule. Table 1 CO electrochemical oxidation mechanisms (7) The microstructure of the cathode is stable and homogeneous. The distributions of the two conducting phases (electronic and CO adsorption CO(g)+(Ni) ←⎯⎯⎯→⎯ CO(Ni) ionic) in the electrodes are assumed to be uniform. O charge transfer In order to simplify the calculation, the three-dimensional domain 2- - O (YSZ)+(Ni) ←⎯⎯⎯→⎯ O(Ni)+(YSZ)+2e shown in Fig. 1 is mapped onto a one-dimensional computational I adsorbed CO reaction domain as shown in Fig.2. In this figure, the model structures, calculation domains and boundaries are labeled schematically, as CO(Ni)+O(Ni) ⎯⎯→ CO (Ni)+(Ni) ←⎯⎯ 2 described later. ⎯⎯→ CO2 desorption CO22 (Ni) ←⎯⎯ CO (g)+(Ni) Is Mechanism I with adsorbed species surface diffusion CO adsorption CO(g)+(Ni) ←⎯⎯⎯→⎯ CO(Ni) CO charge transfer II 2- ⎯⎯→ - CO(Ni)+O (YSZ) ←⎯⎯ CO2 (Ni)+ (YSZ)+2e ⎯⎯→ CO2 desorption CO22 (Ni) ←⎯⎯ CO (g)+(Ni) IIs Mechanism II with adsorbed species surface diffusion The model equations and parameters are described in detail in Section 2. The results are obtained and discussed in Section 3. Finally conclusions are stated in Section 3. 2. Model development Model geometry and assumptions The model assumptions are: Figure 2. Representation of the 3D cell using a 1D calculation domain (1) Gases are modeled as ideal gases. (2) The temperature of the cell is uniform. All parameters are evaluated Using the above assumptions and the simplified model at the given temperature. (3) The electrochemical mechanism is modeled using a set of geometry, a 1D SOFC model is formulated considering elementary reactions that represent chemical reactivity at the anodic heterogeneous chemistry, electrochemistry, charge molecular scale. Heterogeneous thermochemical and and mass balance, as described in the following sections. electrochemical reactions are assumed to take place on both of the Ni surface and YSZ surface. (4) The charge transfer reaction is assumed to be a surface spillover Governing equations reaction taking place at the TPB as shown in Fig. 1. Two different reaction pathways are studied. A. Anode heterogeneous chemistry (5) Surface diffusion is one-dimensional and is modeled as Fickian Heterogeneous chemistry at the catalytic surface of the anode is diffusion along the surface in the direction perpendicular to the used. Ni is an effective catalyst for surface reactions, especially for B. hydrocarbon fueled SOFC. A simplified heterogeneous mechanism 13 14 15 (6) One adsorbed CO molecule occupies one vacant position on the from Hecht et al. , Janardhanan et al. and Zhu et al. is used, as surface, neglecting the possibility of two vacant positions being shown in Table 2. Table 2 Heterogeneous reactions mechanism on the Ni surface a a a -1 Reaction A (cm, mol, s) n E (kJ mol ) Adsorption −02 b 1f O2 + Ni(s) + Ni(s) → O(s) + O(s) 1.000×10 0.0 0.00 −05 b 2f CO2 + Ni(s) → CO2(s) 1.000×10 0.0 0.00 3f CO + Ni(s) → CO(s) 5.000×10−01 b 0.0 0.00 Desorption +23 1b O(s) + O(s) → Ni(s) + Ni(s) + O2 4.283×10 0.0 474.95 +07 2b CO2(s) → CO2 + Ni(s) 6.447×10 0.0 25.98 +11 3b CO(s) → CO + Ni(s) 3.563×10 0.0 111.27 c θCO(s) −50.00 Surface reactions 2 Copyright © 2010 by ASME Downloaded From: https://proceedings.asmedigitalcollection.asme.org on 11/20/2018 Terms of Use: http://www.asme.org/about-asme/terms-of-use 4f C(s) + O(s) → CO(s) + Ni(s) 5.200×10+23 0.0 148.10 4b CO(s) + Ni(s) → C(s) + O(s) 1.354×10+22 −3.0 116.12 c θCO(s) −50.00 +19 5f CO(s) + O(s) → CO2(s) + Ni(s) 2.000×10 0.0 123.60 c θCO(s) −50.00 +23 5b CO2(s) + Ni(s) → CO(s) + O(s) 4.653×10 −1.0 89.32 a Arrhenius parameters for the rate constants are written in the form: k = AT n exp(−E/RT). b Sticking coefficient. c Coverage-dependent activation energy. The surface adsorbates are assumed to be uniformly distributed 0 The sticking coefficient Si is temperature dependent and is expressed over the Ni surface. The species molar production rates depend on the as gaseous species concentrations and the surface species concentrations, 0 b ⎛⎞d which are expressed by the coverage.
Details
-
File Typepdf
-
Upload Time-
-
Content LanguagesEnglish
-
Upload UserAnonymous/Not logged-in
-
File Pages9 Page
-
File Size-