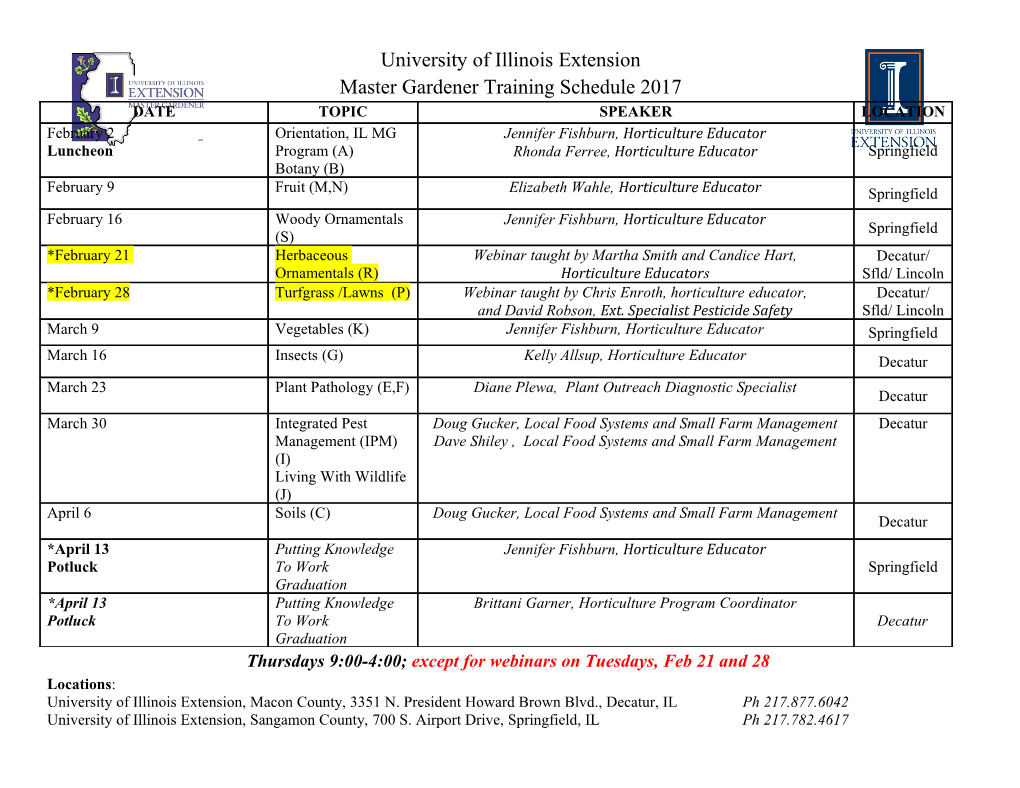
RESEARCH ARTICLE Functional asymmetry and electron flow in the bovine respirasome Joana S Sousa, Deryck J Mills, Janet Vonck, Werner Ku¨ hlbrandt* Department of Structural Biology, Max Planck Institute of Biophysics, Frankfurt, Germany Abstract Respirasomes are macromolecular assemblies of the respiratory chain complexes I, III and IV in the inner mitochondrial membrane. We determined the structure of supercomplex I1III2IV1 from bovine heart mitochondria by cryo-EM at 9 A˚ resolution. Most protein-protein contacts between complex I, III and IV in the membrane are mediated by supernumerary subunits. Of the two Rieske iron-sulfur cluster domains in the complex III dimer, one is resolved, indicating that this domain is immobile and unable to transfer electrons. The central position of the active complex III monomer between complex I and IV in the respirasome is optimal for accepting reduced quinone from complex I over a short diffusion distance of 11 nm, and delivering reduced cytochrome c to complex IV. The functional asymmetry of complex III provides strong evidence for directed electron flow from complex I to complex IV through the active complex III monomer in the mammalian supercomplex. DOI: 10.7554/eLife.21290.001 Introduction Mitochondria are intricate membrane organelles found in virtually all eukaryotic cells, where they serve a number of essential physiological functions. Their central role is to provide energy to the cell in the form of ATP by oxidative phosphorylation. The mitochondrial respiratory chain consists of four complexes (I–IV), which transfer electrons from NADH and succinate to molecular oxygen. A part of *For correspondence: werner. the energy gained in electron transfer is used to pump protons across the inner mitochondrial mem- [email protected] brane. The resulting proton gradient is utilized by the mitochondrial ATP synthase to generate ATP. Competing interest: See The respiratory chain complexes reside mostly, if not entirely, in the mitochondrial cristae (Ku¨hl- page 13 brandt, 2015), which are deep invaginations of the inner membrane into the matrix. NADH:ubiquinone oxidoreductase, also known as complex I, is the largest assembly in the elec- Funding: See page 13 tron transfer chain. Mammalian complex I comprises 44 different subunits, including two copies of Received: 05 September 2016 subunit SDAP, and therefore consists of a total of 45 subunits (Vinothkumar et al., 2014). The 14 Accepted: 03 November 2016 core subunits are conserved from prokaryotes to mammals (Walker, 1992). The characteristic Published: 10 November 2016 L-shape of complex I arises from the association of three different units. The dehydrogenase and Reviewing editor: Stephen C hydrogenase-like units constitute the matrix arm and are responsible for the transfer of electrons Harrison, Harvard Medical from NADH to ubiquinone (Sazanov and Hinchliffe, 2006). As a third unit, the membrane-embed- School, United States ded transporter assembly pumps four protons from the matrix to the cristae lumen per catalytic cycle (Galkin and Terenetskaya, 1999; Leif et al., 1995). Succinate:ubiquinone oxidoreductase, or Copyright Sousa et al. This complex II, is the only complex in the electron transfer chain that does not translocate protons, but article is distributed under the merely feeds electrons into the process. The cytochrome bc complex (complex III) is a symmetrical terms of the Creative Commons 1 Attribution License, which dimer, with cytochrome b, the Rieske iron-sulfur protein and cytochrome c1 as core subunits permits unrestricted use and (Yang and Trumpower, 1986). The Rieske subunit extends across both monomers, stabilizing the redistribution provided that the dimer that is essential for function. In mammals, complex III contains a total of 11 subunits per mono- original author and source are mer, of which eight are supernumerary (Scha¨gger et al., 1986). Complex III transfers electrons from credited. ubiquinone to cytochrome c, a small soluble electron carrier protein in the cristae lumen. Finally, Sousa et al. eLife 2016;5:e21290. DOI: 10.7554/eLife.21290 1 of 17 Research article Biophysics and Structural Biology cytochrome c oxidase, also known as complex IV, transfers electrons from cytochrome c and cata- lyzes the reduction of molecular oxygen to water. Mammalian complex IV has three core subunits (COX1, COX2 and COX3) and 14 subunits in total (Balsa et al., 2012; Kadenbach et al., 1983). The structural organization of the complexes that carry out oxidative phosphorylation in the inner mitochondrial membrane has been subject to numerous investigations. For many years it was assumed that the respiratory chain complexes exist as separate units in the fluid lipid bilayer of the inner membrane and interact by random collision (Hackenbrock et al., 1986). The discovery of supercomplexes by blue-native polyacrylamide gel electrophoresis (BN-PAGE) after solubilization with mild detergents (Scha¨gger and Pfeiffer, 2000) gave rise to the plasticity model, which postu- lates that respiratory complexes can exist both free in the membrane and as larger supramolecular entities (Acin-Perez et al., 2008; D’Aurelio et al., 2006). Since then, several stoichiometric super- complexes have been identified, amongst which the respirasome (supercomplex I1III2IV1) is the most prominent. The respirasome contains all components required to transfer electrons from NADH to molecular oxygen (Scha¨gger and Pfeiffer, 2000). The possible functional and structural roles of supercomplexes have been hotly debated. At the functional level, advantages due to partitioning of the quinol pool and substrate channeling have been postulated and are supported by several independent studies (Bianchi et al., 2003; Lapuente- Brun et al., 2013). Other results are inconsistent with a partitioning of the quinol pool (Blaza et al., 2014). A reduction in the level of reactive oxygen species (ROS) that are generated as side products of electron transfer reactions in the respiratory chain has also been suggested as a possible role (Maranzana et al., 2013; Panov et al., 2006; Seelert et al., 2009). From the point of view of pro- tein structure, supercomplexes have been proposed to confer stability to complex I or assist in its assembly (Marques et al., 2007; Scha¨gger et al., 2004). In line with this, a model for the generation of supercomplexes was proposed, where the assembly of catalytic subunits of the complex I NADH: dehydrogenase module occurs at a late stage to activate the supercomplexes (Moreno- Lastres et al., 2012). However, recent complexome profiling studies failed to detect supercom- plexes containing immature complex I, suggesting that the respirasome forms by association of fully assembled component complexes (Guerrero-Castillo et al., 2016). Several mitochondrial disorders are associated with impaired respirasome formation. Genetic mutations that impair the assembly of complex III result in a loss of complex I and combined com- plex III/I defects (Acin-Perez et al., 2004; Bruno et al., 2003; Lamantea et al., 2002; Scha¨gger et al., 2004). Complex IV deficiencies associated with a reduction of complex I levels in mouse and human cells have also been reported (D’Aurelio et al., 2006; Diaz et al., 2006; Vempati et al., 2009). The secondary loss of complex I upon impaired expression of complexes III and IV has been taken to mean that complex I stability depends on physical interaction with other complexes in the respiratory chain, since pharmacological inhibition was not sufficient to reduce complex I levels to the same extent (Acin-Perez et al., 2004; Diaz et al., 2006). Recent studies sug- gest however that low levels of cytochrome bc1 and cytochrome c oxidase (as well as cytochrome c) result in an accumulation of reduced quinone, which would trigger reverse electron transfer (RET) and generation of superoxide by complex I. This could result in oxidative damage and complex I degradation (Guaras et al., 2016). When ROS production by RET was inhibited, complex I levels were restored (Guaras et al., 2016). Structures of the isolated respirasome at estimated resolutions of 20–30 A˚ have been obtained by negative-stain electron microscopy (EM) (Scha¨fer et al., 2007), single-particle cryo-EM (Althoff et al., 2011) and electron cryo-tomography (Dudkina et al., 2011). However, the map reso- lution was insufficient to fit the component complexes precisely, or to detect new functionally rele- vant features. Moreover, the recently published high-resolution cryo-EM structures of bovine complex I (Vinothkumar et al., 2014; Zhu et al., 2016) contribute to a comprehensive view of the respirasome. Here, we report a cryo-EM map of the supercomplex I1III2IV1 from bovine heart mito- chondria at 9 A˚ resolution. We show specific protein-protein contacts between the three respiratory chain complexes within the respirasome. Our structure reveals a functionally asymmetric complex III, in which one monomer preferentially catalyzes the reduction of cytochrome c in a defined supramo- lecular organization of the electron transport chain. Two cryo-EM studies of the respirasome from ovine and porcine heart mitochondria have been published since this manuscript was submitted (Gu et al., 2016; Letts et al., 2016b). Both are at sig- nificantly higher resolution than our structure, but neither of them observe the functional asymmetry Sousa et al. eLife 2016;5:e21290. DOI: 10.7554/eLife.21290 2 of 17 Research article Biophysics and Structural Biology of complex III, which we regard
Details
-
File Typepdf
-
Upload Time-
-
Content LanguagesEnglish
-
Upload UserAnonymous/Not logged-in
-
File Pages17 Page
-
File Size-