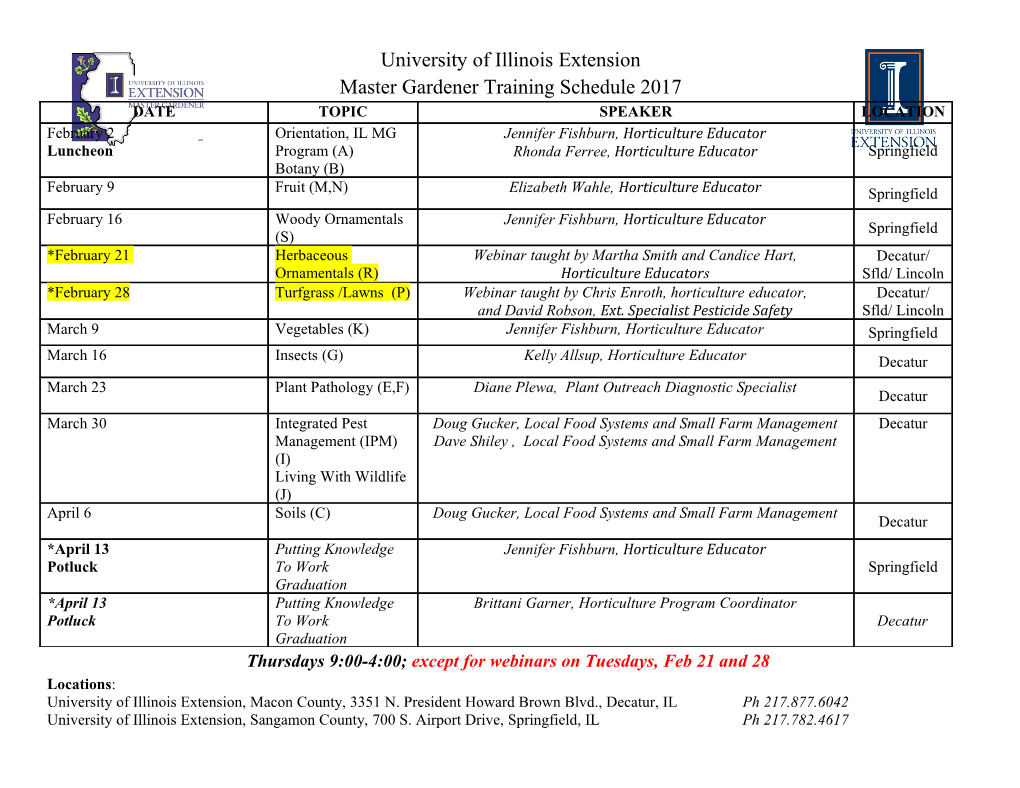
14 Charged Leptons Conveners: B. C. K. Casey, Y. Grossman, D. G. Hitlin J. Albrecht, M. Artuso, K. Babu, R.H. Bernstein, T. Blum, D. N. Brown, B. C. K. Casey, C.-h. Cheng, V. Cirigliano, A. Cohen, A. Deshpande, E. C. Dukes, B. Echenard, A. Gaponenko, D. Glenzinski, M. Gonzalez-Alonso, F. Grancagnolo, Y. Grossman, R.C. Group, R. Harnik, D.G. Hitlin, B. Kiburg, K. Knoepfel, K. Kumar, G. Lim, Z.-T. Lu, D. McKeen, J.P. Miller, M. Ramsey-Musolf, R. Ray, B.L. Roberts, M. Rominsky, Y. Semertzidis, D. Stoeckinger, R. Talman, R. Van De Water, P. Winter 14.1 Executive Summary The enormous physics potential of the charged lepton experimental program was very much in evidence at the Workshop. There are discovery opportunities in experiments that will be conducted over the coming decade using existing facilities and in more sensitive experiments possible with future facilities such as Project X. Exquisitly sensitive searches for rare decays of muons and tau leptons, together with precision measurements of their properties will either elucidate the scale and dynamics of flavor generation, or limit the scale of flavor generation to well above 104 TeV. The crown jewel of the program is the discovery potential of muon and tau decay experiments searching for charged lepton flavor violation (CLFV) with several orders-of-magnitude improvement in sensitivity in multiple processes. There is an international program of CLFV searches, with experiments recently completed, currently running, and soon to be constructed in the United States, Japan, and Europe. These include the completion of the MEG experiment at PSI, an upgrade of MEG, the proposed mu3e search at PSI, new searches from muon to electron conversion (Mu2e at Fermilab, COMET at J-PARC), SuperKEKB, and over the longer term, experiments exploiting megawatt proton sources such as Project X. Over the next decade gains of up to five orders-of-magnitude are feasible in muon-to-electron conversion and in the µ 3e searches, while gains of at least two orders-of-magnitude are possible in µ eγ and τ 3` decay! and more than one order of magnitude in τ `γ CLFV searches. The question of! which of these! processes is the more sensitive was addressed in some! detail at the Workshop; the answer is that the relative sensitivity depends on the type of new physics amplitude responsible for lepton flavor violation. The four-fermion operators that mediate these decays or conversions can be characterized by two parameters, Λ which determines the mass scale of the four fermion amplitude κ, which governs the ratio of the four fermion amplitude and the dipole amplitude. For κ << 1 the dipole-type operator dominates CLFV phenomena, while for κ >> 1 the four-fermion operators are dominant. Figures 14-1 and 14-2, from A. de Gouvea and P. Vogel, Progress in Particle and Nuclear Physics 71, 75 (2013), show these relationships and the capability of new experimental searches, which can extend our knowledge quite dramatically in the next decade. Thus the pattern of violation that emerges thus yields quite specific information about new physics in the lepton sector. Existing searches already place strong constraints on many models of physics beyond the standard model; the contemplated improvements increase these constraints significantly, covering substantial regions of the parameter space of many new physics models. These improvements are important regardless of the outcome of new particle searches of the LHC; the next generation of CLFV searches are an essential 2 Charged Leptons component of the particle physics road map going forward. If the LHC finds new physics, then CLFV searches will confront the lepton sector in ways that are not possible at the LHC, while if the LHC uncovers no sign of new physics, CLFV may provide the path to discovery. 7000 6000 (TeV) (TeV) " " 5000 4000 B(µ # eee)=10-16 3000 B(µ # e conv in 27Al)=10-18 4 -14 10 2000 B(µ # e$)=10 B(µ # e$)=10-13 -14 B(µ # e conv in 27Al)=10-16 B(µ # eee)=10 1000 900 800 700 -14 B(µ # e$)=10 600 B(µ # e$)=10-13 500 400 10 3 300 EXCLUDED (90% CL) EXCLUDED (90% CL) -2 -1 2 -2 -1 2 10 10 1 10 10 10 10 1 10 10 ! ! Figure 14-1. Sensitivity of a µ ! e con- Figure 14-2. Sensitivity of a µ ! eee ex- 27 version in Al experiment27 that can probe a periment that is sensitive to branching ratios 14 Figure 2: Sensitivity of a µ e conversion in Al16 experiment18 that canFigure probe a 3: normalized Sensitivity14 of a µ 16eee experiment that is sensitive to branching ratios 10− normalized16 →18 capture rate of 10 and 10 , and of 16 10 and 10→ , and of a µ ! eγ search that 13 14 capture rate of 10− and 10− , and of a µ eγ search that is sensitiveand to a 10 branching− , and ratio of a µ eγ search that is sensitive to a branching ratio of 10− and 10− , 13 14 → → 13 of 10− and 10−a ,µ to! theeγ newsearch physics that scale is Λ sensitive as a function to a of branchingκ, as definedto the in Eq. new (2). physics Alsois sensitive scale Λ as toa function a branching of κ, as ratiodefined of in10 Eq. (3).and Also depicted is the depicted is the currentlyratio of excluded1013 and region1014 of, to this the parameter new physics space. scalecurrently excluded1014 region, to of the this new parameter physics space. scale Λ as a function Λ as a function of κ, as defined in the text. Also of κ, as defined in the text. Also depicted is depicted is the currently excluded region of this the currently excluded region of this parameter parameter space. κ and Λ if Eq. (3) describesspace. CLFV), studies of electromagnetic properties of charged leptons (g 2, A model independent comparison between the reach of µ eee andelectricµ e dipoleconversion moments), in nuclei precision is studies of neutrino processes (including oscillations), and, of course,− a lot less straight forward. If the new physics is such that the→ dipole-type operator→ is dominant (κ 1 “direct” searches for new, heavy degrees of freedom (Tevatron, LHC). Valuable information, including in Figures 2 andIn 3), general, it is easy muon to see that measurements near-future prospects have the for µthe best naturee sensitivityconversion and chirality searches over of the theare effective largest operators range of that the mediate parameter CLFV, can space be obtained of by observing 16 → comparable to those for µ eee, assuming both can reach the 10− level.µ µe conversione conversion in different searches nuclei [14, 29, 30] or by studying the kinematical distribution of the many new→ physics models. There are,17 however, models→ in which rare tau decays could provide the discovery will ultimately dominate, assuming these can reach beyond 10− , andfinal-state→ assuming electronsµ eee in searchesµ eee (see [14] and references therein). “saturate” at thechannel. 10 16 level.τ Underflavor all violation other theoretical searches circumstances, will have keeping their in sensitivity mind→ that κ →and extended Λ by around an order of magnitude at − + − Before moving on to specific new physics scenarios, it is illustrative to compare, as model-independently in Eqs. (2,3) arenewnot thee same,e flavor it is impossible factories. to unambiguously Polarized electron compareas possible,beams the two CLFV cannew physicsprovide probes. that an mediates additional CLFV gain and the in sensitivity. new physics that It wasmay haveclear manifested itself in The discussionsfrom above the also discussion serve to illustrate that as another many “feature” different ofprecision searches CLFV measurements for searches CLFV violation. as of feasible the muon should anomalous be magnetic conducted, moment. since In athe nutshell, best the world’s most In the case of adiscovery positive signal, channel the amount is model-dependent of information regarding and theprecise the new model measurement physics is is not limited. of yet the Forg known.2 of the Should muon disagrees a signal with be the observed world’s best in Standard any Model estimate − example, a positivechannel, signal in searches a µ e conversion and measurements experiment does in not as allow manyfor this one CLFV observableto measure channels at either theΛ 3 as.6 orσ possiblelevel (for willan updated be crucial overview to determiningsee [1], and references the therein). New, κ but only a function of the two.→ In order to learn more about the newheavy physics, physics one needs contributions to combine to the muon g 2 are captured by the following effective Lagrangian: − information involvingnature the rateof the of aunderlying particular CLFV physics, process with since other correlations observables. Thesebetween include the other rates expected in different channels provide mµ µν CLFV observablesa powerful (e.g., a positive discriminator signal in µ betweeneγ and µ physicseee would model. allow one to measure both g 2 µ¯RσµνµLF + h.c. (4) → → L − ⊃ Λ2 The new muon g 2 experiment7 will measure the anomaly to close to 100 parts8 per billion precision with different experimental− techniques. This will be an important measurement whether or not the LHC sees new physics. If the LHC sees SUSY-like new physics, g 2 will be used as a constraint in determining which model we see. The LHC will be particularly sensitive to− color super-partners, while g 2 can pin down the flavor sector. The sensitivity of g 2 to tan β will provide a test the universality of that− parameter. If the LHC does not see new physics, then− g 2 can be used to constrain other models, such as theories involving − Community Summer Study: Snowmass 2013 14.2 Overview 3 dark photons and extra dimensions.
Details
-
File Typepdf
-
Upload Time-
-
Content LanguagesEnglish
-
Upload UserAnonymous/Not logged-in
-
File Pages42 Page
-
File Size-