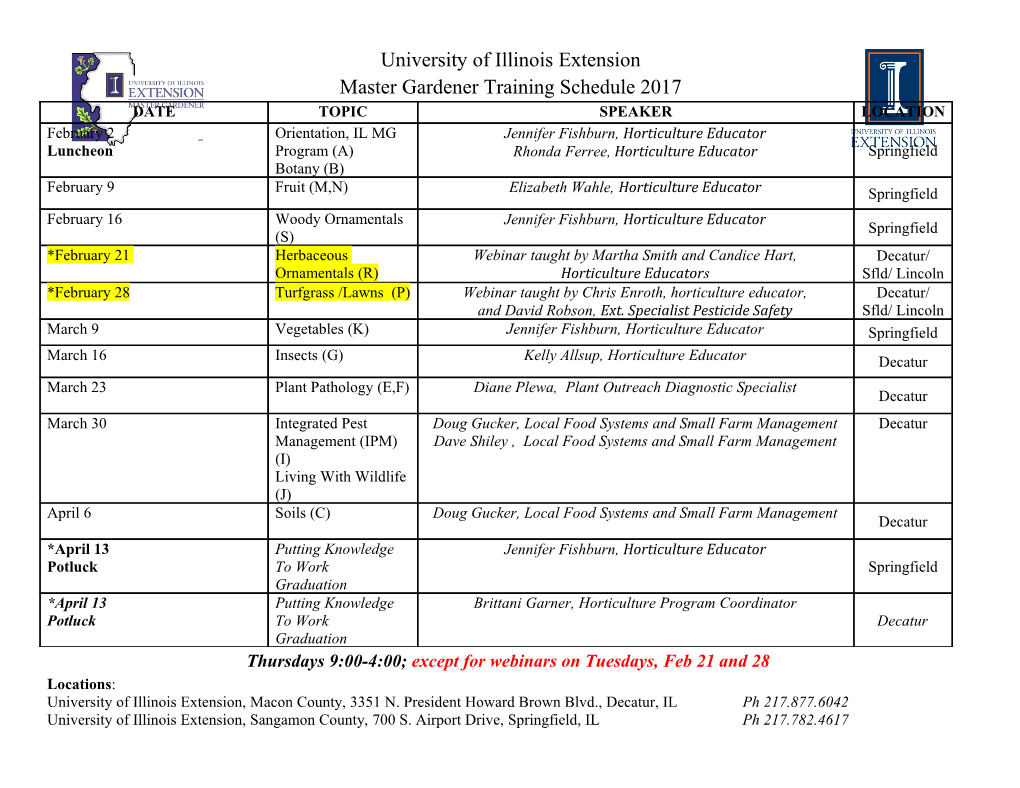
1 The importance of geologists and geology in tsunami 2 3 science and tsunami hazard 4 5 DAVID R. TAPPIN 6 7 British Geological Survey, Keyworth, Nottingham, NG12 5GG, UK, 8 [email protected] 9 10 11 Abstract: Up until the late 1980s geology contributed very little to the study of tsunamis because 12 most were generated by earthquakes which were mainly the domain of seismologists. In 1987–88 13 however, sediments deposited as tsunamis flooded land were discovered. Subsequently they began to be widely used to identify prehistorical tsunami events, providing a longer-term record than pre- 14 viously available from historical accounts. The sediments offered an opportunity to better define 15 tsunami frequency that could underpin improved risk assessment. When over 2200 people died 16 from a catastrophic tsunami in Papua New Guinea (PNG) in 1998, and a submarine landslide 17 was controversially proven to be the mechanism, marine geologists provided the leadership that 18 led to the identification of this previously unrecognized danger. The catastrophic tsunami in the 19 Indian Ocean in 2004 confirmed the critical importance of sedimentological research in under- 20 standing tsunamis. In 2011, the Japan earthquake and tsunami further confirmed the importance 21 of both sediments in tsunami hazard mitigation and the dangers from seabed sediment failures 22 in tsunami generation. Here we recount the history of geological involvement in tsunami science and its importance in advancing understanding of the extent, magnitude and nature of the hazard 23 from tsunamis. 24 25 26 27 28 Until the late 1980s tsunami science was mostly The involvement of geologists in tsunami 29 the province of seismologists, numerical modellers, research began in the early 1980s, with the first 30 geophysicists and historians; tsunamis received papers on deep-sea deposits in the Aegean Sea. 31 little attention from geologists (e.g. Bailey & Weir Here, unusual seabed sediments, termed homoge- 32 1933; Coleman 1968, 1978). Earthquakes were nites, were proposed as deposited from a tsunami 33 considered the main, if not the only, mechanism generated by the Late Bronze Age eruption of San- 34 that could generate elevated tsunami waves that torini (Kastens & Cita 1981; Cita et al. 1984). In the 35 were very destructive at the coast. Other tsunami- Hawaiian Islands, boulders and coarse-grained 36 generation mechanisms, such as submarine land- gravels preserved at high elevations (hundreds of 37 slides, were not considered a major hazard despite metres) were considered to result from tsunamis 38 evidence to the contrary such as from the Grand generated by large-scale volcanic collapse (Moore 39 Banks event of 1929 (Bardet et al. 2003). Numerical & Moore 1984). The early results from the Mediter- 40 tsunami modelling of submarine landslides was in ranean and Pacific were controversial due to the 41 large part theoretical (Jiang & LeBlond 1992, uncertainty over whether there were tsunamis gen- 42 1994). The slow landslide failure velocities were erated at these locations. In addition, this approach 43 perceived as inhibiting tsunami generation, in of using sediments to identify tsunamis from their 44 contrast to earthquake-generated tsunamis where sedimentary record had not been attempted before. 45 rupture velocities of kilometres per second were The tsunami from the Late Bronze Age (LBA) erup- 46 regarded as instantaneous in the context of the tion of Santorini had been a major controversy for 47 relatively slow (metres per second) velocity of tsu- decades (Marinatos 1939). Sand had been found 48 nami wave generation (Geist 2000; Ward 2001). in Minoan buildings on the coast and used as evi- 49 Historians provided evidence on older tsunamis, dence for tsunami inundation; its origin was how- 50 hopefully for use in estimating tsunami fre- ever disputed as it was located at sea level and the 51 quency–magnitude relationships for future tsunami sand could have been deposited from storms or 52 risk, although the limitations of historical data were was present for religious purposes. Subsequent 53 recognized (Ambraseys & Jackson 1990). When a validation has been impossible because the sand 54 paper (rarely) considered the geology of tsunamis, deposits were destroyed during subsequent excava- 55 it was on the sediments deposited from inundation tions. The Aegean Sea homogenites provided the 56 and authored by seismologists (e.g. Wright & first geological evidence in support of the tsunami 57 Mella 1963). hypothesis but, even today, the origin of the 58 From:Scourse, E. M., Chapman, N. A., Tappin,D.R.&Wallis, S. R. (eds) Tsunamis: Geology, Hazards and Risks. Geological Society, London, Special Publications, 456, https:// doi.org/10.1144/SP456.11 # British Geological Survey@ NERC. Published by The Geological Society of London. All rights reserved. For permissions: http:// www.geolsoc.org.uk/permissions. Publishing disclaimer: www.geolsoc.org.uk/pub_ethics D. R. TAPPIN 59 homogenites remains controversial (see Pareschi determine tsunami characteristics (Jaffe et al. EDQ1 60 et al. 2006; Shanmugam 2006; Weiss 2008; Polonia 2007). More recent, devastating, events in the Indian 61 et al. 2013). The suggestion that the Hawaiian Ocean (2004) and Japan (2011) have expanded 62 deposits were from tsunamis was groundbreaking this geological involvement in tsunami sediment 63 because of their use in identifying tsunamis from characterization (e.g. Paris et al. 2007), in inverse 64 volcanic collapse. Previously, the deposits were modelling of tsunami-generation mechanisms 65 interpreted as laid down during sea-level highstands (Spiske et al. 2010; Sugawara et al. 2014) and in 66 (Stearns 1978). Their origin is also still disputed research on submarine landslides in tsunami gener- 67 (Stearns 1978; Rubin et al. 2000; McMurtry et al. ation (Tappin et al. 2007, 2014). Storegga, PNG 68 2004a). and most recently Japan have led to an increased 69 In 1987 and 1988, two groundbreaking papers realization of the tsunami hazard from submarine 70 published on tsunami sediments demonstrated landslides. 71 their potential in evaluating tsunami hazard. The Here it is recounted how over the past c. 30 72 first (Atwater 1987) was on prehistoric sediments years geologists became increasingly involved in 73 in Cascadia, which identified a sequence of earth- tsunami science and how they have contributed to 74 quakes and their associated tsunamis that extended an improved understanding of tsunami mechanisms 75 back in time to over 10 ka before present (BP). and their hazard. Although there were earlier, iso- 76 The second paper (Dawson et al. 1988) on sedimen- lated precursors to the main ‘catalyst’ events iden- 77 tary deposits in Scotland identified a major prehis- tified above, it was during the 1980s that ‘geology’ 78 toric tsunami from a massive submarine landslide became significant in advancing tsunami science; 79 (Storegga) located off Norway (Bugge 1983; this advancement was initially from the application 80 Bugge et al. 1988). These sediments motivated the of tsunami sediments, followed by an improved 81 first attempt at numerical modelling of a tsunami understanding of tsunami frequency, hazard and 82 from a submarine landslide mechanism (Harbitz risk, and more recently, with the Japan tsunami, 83 1992). In 1998, a devastating tsunami struck the from the use of sediments as a basis for inverse 84 north coast of Papua New Guinea (PNG) killing numerical models of tsunami-generation mecha- 85 over 2200 people (Kawata et al. 1999). The associ- nisms (e.g. Sugawara et al. 2014). The motivations 86 ated Mw 7 magnitude earthquake was too small to for this paper were the two meetings held in Japan 87 generate all of the elevated local run-ups of 15 m. and the United Kingdom in 2014 and 2015 on the 88 Amid confusion and controversy (e.g. Geist 2000), theme of ‘Tsunami Hazards and Risks: Using the 89 marine surveys organized in response to the disaster Geological Record’. The focus of the Japan meet- 90 acquired hydroacoustic and sample data offshore ing was the use of tsunami sediments in the mitiga- 91 of the impacted area. These surveys identified an tion of tsunami hazard. The aim of the UK meeting 92 offshore slump, which preliminary numerical simu- was to bring together geologists and hazard and 93 lations demonstrated to be the local tsunami mech- risk modellers. In this paper the use of the geolog- 94 anism (Tappin et al. 1999). The PNG event was ical record in contributing to tsunami hazard is 95 seminal in identifying the major hazard from sub- extended by the addition of how the identification 96 marine landslides in tsunami generation. It was the of submarine landslides have led to their recogni- 97 first tsunami to be studied from responsive marine tion as a major hazard in tsunami generation, 98 surveys led by geologists. Submarine landslides a hazard previously overlooked (Bardet et al. 99 were well researched previously, but rarely in the 2003; Løvholt et al. 2015). To underpin realistic 100 context of tsunami generation and not by collabora- modelling of tsunamis generated from submarine 101 tion between geologists and numerical modellers landslides requires their architectures to be deter- 102 (e.g. Grand Banks in 1929). mined from hydroacoustic data, including multi- 103 The 1987–88 research on tsunami sediments in beam echosounders (MBES) and seismics. 104 the USA and Europe, together with the landslide- Mapping of seabed failures has led to the transition 105 generated PNG tsunami, resulted in the major from theoretical numerical models, which sug- 106 involvement of geologists in tsunami science and gested that landslides were ineffective in tsunami 107 the recognition of the contribution which geology generation (LeBlond & Jones 1995), to realistic, 108 could make to an improved understanding of tsuna- event-based models that prove otherwise (Harbitz 109 mis and their hazard. Demonstrating the mechanism 1992; Tappin et al. 1999).
Details
-
File Typepdf
-
Upload Time-
-
Content LanguagesEnglish
-
Upload UserAnonymous/Not logged-in
-
File Pages34 Page
-
File Size-