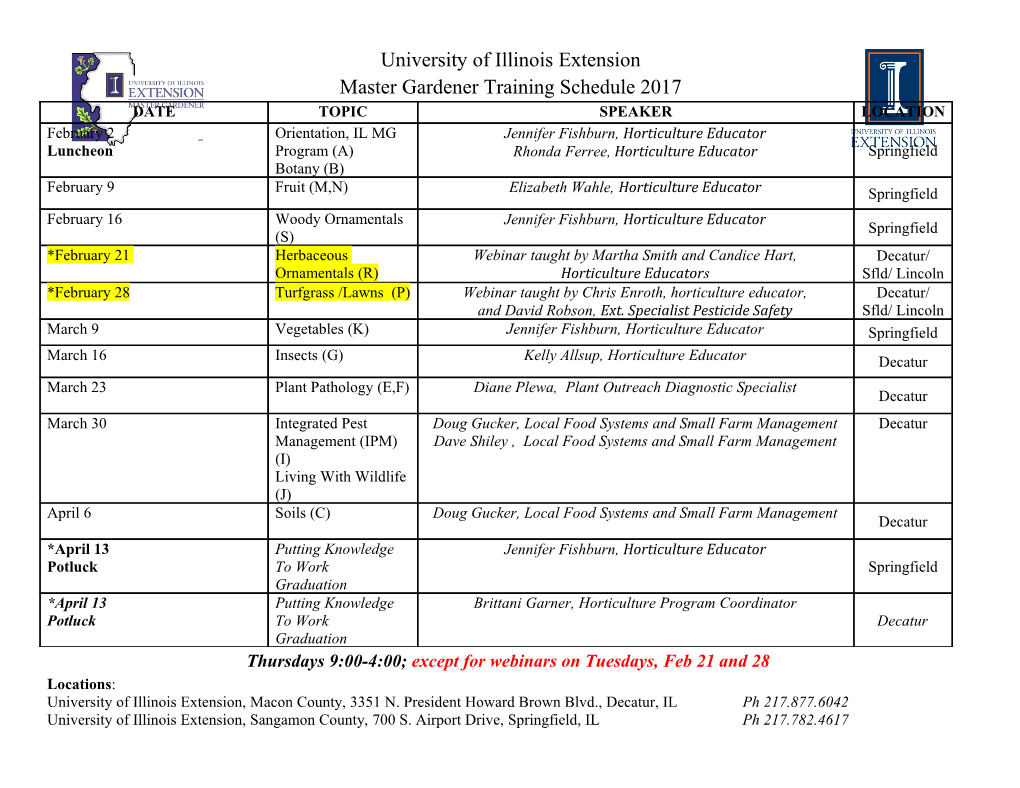
2017 3rd International Conference on Applied Mechanics and Mechanical Automation (AMMA 2017) ISBN: 978-1-60595-479-0 Mechanism Study on the Preparation of Dimethyldichlorosilane Catalyzed by γ-Al2O3 Wen-yuan XU1,*, Xi KUANG1, Zhi-li FANG1, Zan-ru GUO1, Shao-ming YANG1 and San-guo HONG2 1School of Materials Science and Engineering, East China Jiaotong University, Nanchang 330013, P.R. China 2College of Chemistry, Nanchang University, Nanchang 330013, P.R. China *Corresponding author Keywords: Disproportionation, Dimethyldichlorosilane, MP2, DFT, γ-Al2O3. Abstract. Disproportionation method for the preparation of dimethyldichlorosilane can effectively solve a series of problems caused by the accumulation of by-products generated by direct synthesis. This method can turn wastes into treasures but the mechanism is still unknown. The B3LYP/6-31G and MP2/6-311++ G(3df, 2pd) methods were used to calculate the mechanism of the disproportionation catalyzed by γ-Al2O3. The results showed that the active center of the reaction system of γ-Al2O3 is proton. The first step and the fifth step were the rate-limiting step of main reaction and side reaction respectively, which the activation energy barrier is 151.60 and 389.25 (kJ•mol-1) respectively. By comparing the activation energy values, it was found that the reaction path which produce the main product dimethyldichlorosilane could proceed smoothly, and thus matches the results of the experiments. Introduction Silicone materials become the focus of major industrial concerns quickly because of its excellent performance, and the production of organic silicon monomer, especially the dimethyldichlorosilane (M2), is essential for the silicone industry[1]. But the growing content of the by-products including methyltrichlorosilane (M1) and trimethylchlorosilane(M3) produced by direct synthesis is becoming a big issue to be solve, for that long-term storage or direct emission will cause tank corrosion or environment pollution. Therefore, the question that how to achieve the preparation of M2 using the disproportionation reaction of by-product (M1 and M3) has caused great attention. The catalyst of the disproportionation reaction to turn wastes into treasures has also been a research focus for scientists. The choice of catalyst has a significant effect on the disproportionation reaction[2-4]. Owing to the performances of uniform particle size distribution, high purity and activity, excellent [5] dispersion, large specific surface area and high-temperature resistance , γ-Al2O3 not only has exhibited promising applications in catalytic field[6], but also is cheap and conductive to industrialization promotion. In the general catalytic reaction, γ-Al2O3 is not directly involved in the chemical reaction of the catalytic process but play a role in dilution, support and dispersion of [7] precious metals . However, γ-Al2O3 itself is a good catalyst with strong adsorption and Lewis acidic [8] [9] center on surface , and it can improve the conversion and yield effectively . γ-Al2O3 catalyst can achieve the preparation of M2 in the disproportionation reaction, and a series of previous experiments confirmed its good catalytic performance[10]. The results showed that the catalytic performance of γ-Al2O3 was the best in five types of Al2O3 (α, β, θ, γ and η), and the maximum yield of M2 is 24.2% at 433.15K[10]. At present, the experimental study of the preparation of M2 catalyzed by γ-Al2O3 using M1and M3 has been carried out fully, but its mechanism is not clear, including its active center. It is necessary to study the catalytic mechanism of γ-Al2O3 and provide some theoretical basis for the experiments. 327 Computational Methods and Model The reactants, products and intermediates of each reaction have been calculated at the B3LYP level of density functional theory[11,12] with 6-31G basis set using Gaussian 09W program package. Each optimum geometry has been obtained along with the vibration frequency. The geometries for transition states were searched by TSN method. And the transition states are certificated through vibration analysis and calculated through intrinsic reaction curve(IRC), because transition states were characterized by one imaginary frequency for the saddle point[13]. All the energies of the optimized structures were recalculated by the secondary perturbation MP2/6-311++ G(3df, 2pd) method, considering Zero Point Energy (ZPE). As was shown in Fig.1, the following construction unit was intercepted from the γ-Al2O3 lattice structure by use of the Materials Studio software package[5] for theoretical study. The stretch vibration frequency of O-H obtained by structure optimization and frequency calculation is 3676cm-1, and this theoretical data is only 1.6% away from the experimental results, which is reported to be 3618cm-1[14]. The data within the accepted error range demonstrated that the segment structure of γ- Al2O3 is a right model for simulation and study of acid sites. Figure 1. Cubic Stacking Figure Of γ-Al2O3 in materials studio and optimized model of γ-Al2O3 and its key parameters. (bond length: nm; bond angle:). Figure 2. The reaction processes of the dispropportionation reaction catalyzed by γ-Al2O3. The stable structure and important geometric parameters of the γ-Al2O3 model are shown in Fig 1. The model of stable γ-Al2O3 optimized consists of three four-membered rings (Al-O-Al-O) and the central Al atom is bonding to six O atoms. The structure of the model is very regular, almost symmetrical, as evidenced by the bond lengths of 6 Al-O and the three bond angles of O-Al-O, which are almost the same. H atoms were used to saturating the O atoms which were cut out for the model of γ-Al2O3. Thus each O atom was connected with proton and formed acid cites. Previous study have shown that the proton hydrogen is indeed provided by H on the bridge oxygen[15]. This also verifies that the model structure of γ-Al2O3 in Figure 1 is credible. The mechanism of disproportionation was discussed in the proton acid catalytic reaction. 328 Results and Discussion Considering the orders of adsorption and desorption of methyltrichlorosilane (M1) and trimethylchlorosilane (M3) on γ-Al2O3, the reaction is carried out in two pathways, shown in Fig 2. In both pathways, each transition state (TS) of the six has the trends of generating the products (Fig. 3) and restoring the reactants (figure omitted). The numbers of key atoms are shown in Fig 3. Path A: In the first step, the γ-Al2O3 catalyst and M3 form an absorption compound (named R1, similarly hereinafter), then go through TS1 to produce its product P1, with active energy Ea1. The 3 active H atom on the oxygen bridge move close to the -CH3 of M3, and produces CH4. And the Si atom and O2 atom move close to each other at the same time, generating product P1, as is shown in Fig. 3(a). In the second step, M1 reacted to the intermediate contained in product P1, and formed R2, then go through the transition state TS2 and produce P2. The Cl2 atom is supplied by M1 and move close to the Si3 atom which is supplied by the intermediate. And then M2, the main product we need, is produced. Meanwhile, the Si4 atom move close to and bond to the O1 atom, generating product P2(Fig. 3(b)). In the third step, the intermediate contained in product P2 formed R3 with the CH4 which is produced in the first step, and go through TS3 and produce P3. CH4 provide the -CH3 group, and Si4 atom move close gradually to produce M2, the main product. At the same time, H1 1 atom in the CH4 and O atom close to each other restoring the catalyst γ-Al2O3, as is shown in Fig. 3(c). We obtained M2 through the last two steps of this path. Path A is the main reaction of the disproportionation process. Path B: In the first step, the γ-Al2O3 catalyst and M1 form R1, then go through TS4 to produce its product P4, with active energy Ea4. In Fig. 3(d), the C2 atom in M1 is gradually away from the Si4 atom, then moves close and bonds to the H1 atom which was on the oxygen bridge originally. As the oxygen bridge is broken, the Si4 atom attacks the O1 atom, till the atomic distance is shorten enough to bond. And the product P4 is produced. The formation of R5 is in the second step of the side 4 reaction, where M3 is absorbed by the intermediate in P4. SiCl4 is taken off when the Si atom bonds to the Cl2 atom in M3, and the O1 atom in the oxygen bridge move close to Si3 atom in the meantime, generating the intermediate of P5. In the third step, the intermediate contained in product P5 forms R6 with the CH4 which is produced in the first step, then go through TS6 and produce P6. 1 1 The H atom in the CH4 and O atom close to each other restoring the catalyst γ-Al2O3, as is shown in Fig. 3(f). The by-product had been produced through this path. Path B is the side reaction of the disproportionation process. (a) (b) (c) (d) (e) (f) Figure 3. The vibration models and key atom number of transition states generating the products. 329 Figure 4. The spacing trends of key atoms along irc in the catalytic system of γ-Al2O3. It can be seen from Fig 2 that the active center of the disproportionation is proton. H atom achieve the entire disproportionation reaction process from supplying proton for participating in the reaction to reducing proton H on bridge oxygen while restoring the catalyst. To prove that the two reaction paths are credible, the changes of distances between the key atoms in the spacing during the reaction were calculated and shown in Table 1.
Details
-
File Typepdf
-
Upload Time-
-
Content LanguagesEnglish
-
Upload UserAnonymous/Not logged-in
-
File Pages6 Page
-
File Size-