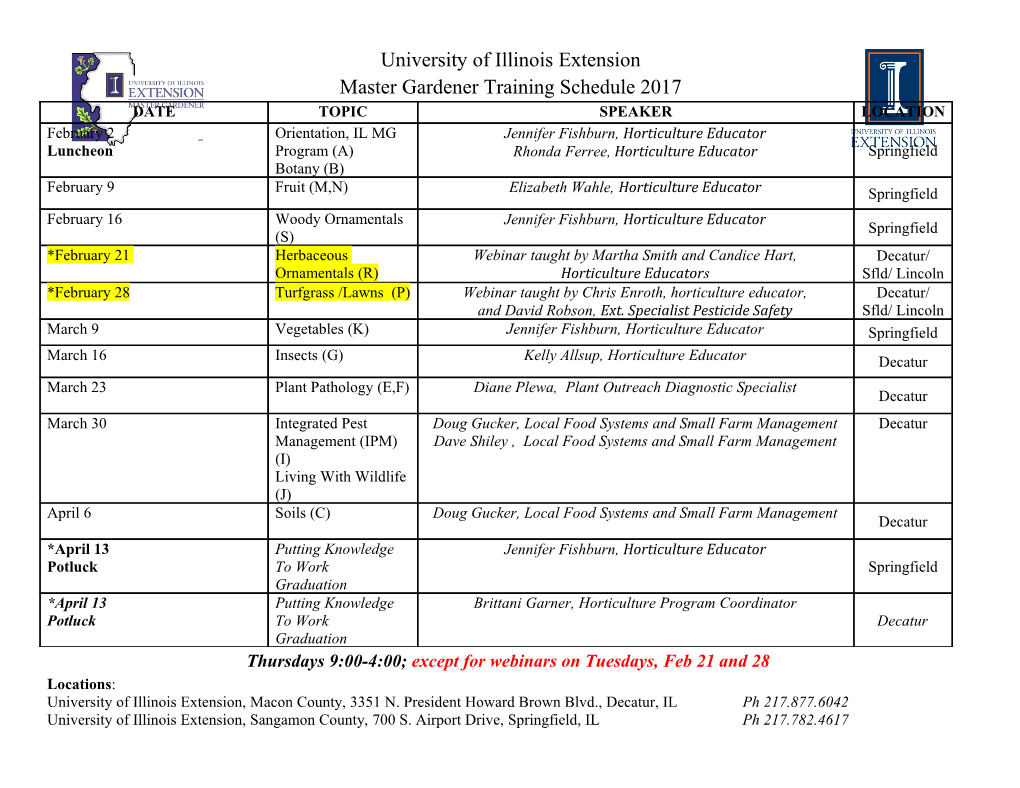
Vol 465 | 6 May 2010 | doi:10.1038/nature09024 LETTERS Designed biomaterials to mimic the mechanical properties of muscles Shanshan Lv1, Daniel M. Dudek2{, Yi Cao1, M. M. Balamurali1, John Gosline2 & Hongbin Li1 The passive elasticity of muscle is largely governed by the I-band To engineer titin-mimicking artificial elastomeric proteins (see part of the giant muscle protein titin1–4, a complex molecular spring Methods and Supplementary Fig. 1), we used the well-characterized composed of a series of individually folded immunoglobulin-like GB1 domains12 to mimic folded titin immunoglobulin domains, domains as well as largely unstructured unique sequences5. These because GB1 domains exhibit mechanical properties comparable to mechanical elements have distinct mechanical properties, and when those of titin immunoglobulin domains12, and we used a consensus combined, they provide the desired passive elastic properties of repeat of the random-coil-like protein resilin13 to mimic unstruc- muscle6–11, which are a unique combination of strength, exten- tured sequences (such as the N2B sequence in titin), because resilin sibility and resilience. Single-molecule atomic force microscopy is a highly elastic and resilient protein13,18,19 and is also largely (AFM) studies demonstrated that the macroscopic behaviour of unstructured20 (see Supplementary Fig. 2). With these two building titin in intact myofibrils can be reconstituted by combining the blocks, we constructed artificial elastomeric proteins (G–R)4 and mechanical properties of these mechanical elements measured at GRG5RG4R, where G represents individual GB1 domain, and R the single-molecule level8. Here we report artificial elastomeric represents individual resilin repeat. proteins that mimic the molecular architecture of titin through We first used AFM techniques6,21 to characterize their nanomecha- 12 the combination of well-characterized protein domains GB1 and nical properties at the single-molecule level. Stretching (G–R)4 resilin13. We show that these artificial elastomeric proteins can be results in characteristic sawtooth-like force–extension relationships12 photochemically crosslinked and cast into solid biomaterials. These (Fig. 1a), where individual force peaks correspond to the mechanical biomaterials behave as rubber-like materials showing high resili- unfolding of GB1 domains and are characterized by an unfolding ence at low strain and as shock-absorber-like materials at high force of ,180 pN and a contour length increment DLc of ,18 nm. strain by effectively dissipating energy. These properties are com- Owing to the dimerization of (G–R)4 via carboxy-terminal cysteine parable to the passive elastic properties of muscles within the residues (Supplementary Information), force–extension curves can physiological range of sarcomere length14 and so these materials show as many as eight GB1 unfolding events. The featureless ‘spacer’, represent a new muscle-mimetic biomaterial. The mechanical which is of length L0 and occurs before the GB1 unfolding force properties of these biomaterials can be fine-tuned by adjusting peaks, corresponds to the stretching of random-coil-like resilins the composition of the elastomeric proteins, providing the oppor- and folded GB1 domains (Fig. 1a), confirming the entropic spring- tunity to develop biomaterials that are mimetic of different types of nature of resilin repeats. Because the persistence length of GB1 is muscles. We anticipate that these biomaterials will find applica- much larger than that of unstructured resilin, fitting the Worm- tions in tissue engineering15 as scaffold and matrix for artificial like-chain model of polymer elasticity to the spacer yielded a persist- muscles. ence length of 0.49 6 0.09 nm (average 6 s.d., n 5 188) for resilin, The string of folded immunoglobulin domains and unstructured comparable to that of the random-coil-like sequence N2B in titin8,9,22 unique sequences constitute two distinct types of entropic springs in and unfolded polyprotein chains6,21. Stretching polyprotein 7,8 titin . The string of folded immunoglobulin domains has higher per- GRG5RG4R yielded force–extension curves with similar sawtooth sistence length than the unstructured sequences and extend first during patterns but with shorter spacers owing to the fewer resilin domains stretching. Only under high stretching forces at the high end of the in GRG5RG4R (Fig. 1b and Supplementary Fig. 3). Moreover, the physiological range of sarcomere length, when the string of immuno- mechanical unfolding of GB1 domains is reversible, because globulin domains are straightened, can a small number of folded unfolded GB1 domains can refold to regain mechanical resistance 12 immunoglobulin domains unfold to extend the length of titin and upon relaxation . These nanomechanical properties of (G–R)4 and 16 dissipate energy, effectively preventing damage due to overstretching . GRG5RG4R largely mimic those of individual titin molecules. These features are combined to give rise to the passive mechanical We then used these miniature-titin-like elastomeric proteins to properties of muscles at the macroscopic level1,2,7,17, which manifest construct biomaterials to mimic the passive mechanical properties as a Young’s modulus close to 100 kPa, increasing energy dissipation at of muscle. Individual titin molecules are well-aligned and organized higher sarcomere length, and stress relaxation at a constant strain4,14,16. in the filament lattice of muscle3. However, it remains challenging to To design biomaterials mimicking the fine-tuned passive elastic pro- mimic such ordered structures in synthetic biomaterials. As an perties of muscle, it is critical to incorporate these mechanical features alternative, we created chemically crosslinked GB1–resilin networks at both single-molecule and macroscopic biomaterial levels. Towards to exploit the nanomechanical properties engineered into individual 21 this goal, here we first engineered artificial elastomeric proteins that GB1–resilin molecules. We used the well-developed [Ru(bpy)3] - mimic the molecular architecture and nanomechanical properties of mediated photochemical crosslinking strategy23, which allows the individual titin molecules. Then we used these proteins to construct crosslinking of two tyrosine residues in close proximity into dityrosine biomaterials that mimic the passive elastic properties of muscle. adducts (Supplementary Fig. 4). This method was used successfully to 1Department of Chemistry, University of British Columbia, Vancouver, British Columbia V6T 1Z1, Canada. 2Department of Zoology, University of British Columbia, Vancouver, British Columbia V6T 1Z1, Canada. {Present address: Department of Engineering Science and Mechanics, Virginia Polytechnic Institute and State University, Blacksburg, Virginia 24061, USA. 69 ©2010 Macmillan Publishers Limited. All rights reserved LETTERS NATURE | Vol 465 | 6 May 2010 a R RRR Resilience is a measure of a material’s ability to deform reversibly GB1 GB1 GB1 GB1 without loss of energy18. Resilin is known for its superb resilience19, ΔL c = 18.3 nm and resilin-based biomaterials constructed using the same photoche- mical crosslinking method did not show appreciable hysteresis even 13,31 200 pN at 250% strain (Fig. 2d). To examine the influence of folded GB1 domains on the resilience of GB1–resilin-based biomaterials, we measured the resilience of these biomaterials. The stretching and relaxation curves of both (G–R)4 and GRG5RG4R at low strain (,15%) were superimposable and no hysteresis was observed (Fig. 2b, c), suggesting high resilience for both materials at low strains. However, the stretching and relaxation curves were no longer superposable at higher strains and hysteresis started to develop, indi- Force (pN) Force cating that some of the work done during stretching was dissipated b R RR GB1 GB1 GB1 GB1 GB1 GB1 GB1 GB1 GB1 GB1 and cannot be recovered upon relaxation. The hysteresis increases L 0 with the increase of strain (Fig. 2b, c), indicating that the resilience of GB1–resilin-based materials decreases with the increase of strain (Fig. 2d). This behaviour is similar to that of myofibrils or myo- cytes14,16,32, which showed increasing hysteresis between stretching and relaxation at increasing sarcomere lengths, indicating that GB1–resilin-based biomaterials, just like muscles16, behave like shock-absorbers at higher strains by effectively dissipating energy. The observed hysteresis, that is, energy dissipation, during cyclic 0 50 100 150 200 250 experiments indicates that stretching GB1–resilin-based biomaterials Extension (nm) to higher strains involved the breakage of weak non-covalent bonds33 in Figure 1 | Force–extension curves of two polyproteins. a,(G–R). the crosslinked network. And the breaking of such bonds is reversible, 4 as the hysteresis observed in GB1–resilin-based biomaterials can be b,GRG5RG4R. The force peaks, characterized by a DLc of ,18 nm and an unfolding force of ,180 pN, result from the mechanical unfolding of GB1 fully recovered upon relaxation. As shown in the insets of Fig. 2b and domains. Stretching resilins does not result in any unfolding force peaks; c, during subsequent stretching, stress–strain curves superpose on one instead we see a featureless spacer of length L0.Thenotabledifferencebetween another regardless of the final strain, suggesting a full recovery of the the force–extension curves of (G–R)4 and GRG5RG4R is the shorter featureless hysteresis. Moreover, the recovery of hysteresis is very fast: during
Details
-
File Typepdf
-
Upload Time-
-
Content LanguagesEnglish
-
Upload UserAnonymous/Not logged-in
-
File Pages5 Page
-
File Size-