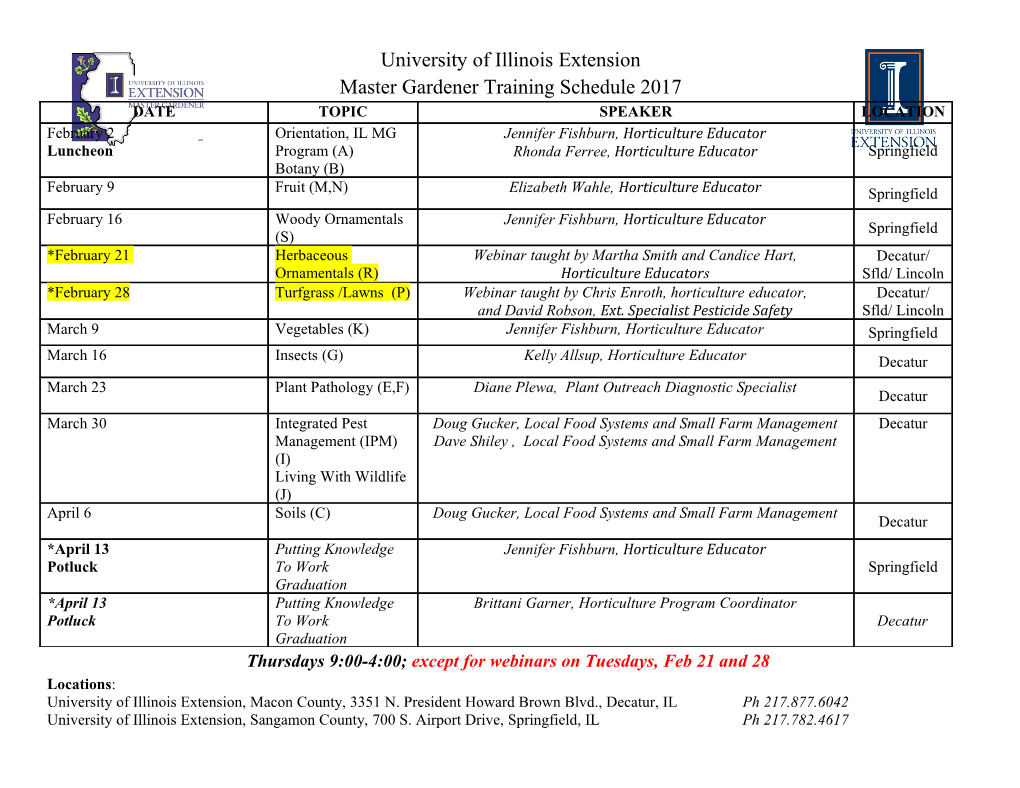
On the Fraction of Internal Tide Energy Dissipated Near Topography Louis C. St. Laurent Department of Oceanography, Florida State University, Tallahassee, Florida Jonathan D. Nash College of Oceanic and Atmospheric Science, Oregon State University, Corvallis, Oregon Abstract. Internal tides have been implicated as a major source of me- chanical energy for mixing in the ocean interior. Indeed, microstructure and tracer measurements have indicated that enhanced turbulence levels occur near topography where internal tides are generated. However, the details of the energy budget and the mechanisms by which energy is transferred from the internal tide to turbulence have been uncertain. It now seems that the energy levels associated with locally enhanced mixing near topography may constitute only a small fraction of the available internal tide energy flux. In this study, the generation, radiation, and energy dissipation of deep ocean internal tides are examined. Properties of the internal tide at the Mid-Atlantic Ridge and Hawaiian Ridge are considered. It is found that most internal tide energy is generated as low modes. The Richardson number of the generated internal tide typically exceeds unity for these motions, so direct shear instability of the generated waves is not the dominant energy transfer mechanism. It also seems that wave-wave interactions are ineffective at transferring energy from the large wavelengths that dominate the energy flux. Instead, it seems that much of the generated energy radiates away from the generation site in low mode waves. These low modes must dissipate somewhere in the ocean, though this likely occurs over O(1000 km) distances as the waves propagate. Additionally, a small fraction of energy flux is generated as high mode waves. These have slower group speeds, higher shear, and faster wave-wave interaction rates than the low modes, and so are likely to dissipate as turbulence close to the topography of the generation site. Preliminary estimates suggest that turbulent dissipation near sites of generation accounts for 30% or less of the total generated energy flux. Based on these estimates, a parameterization of the mixing sustained by high mode internal tide generation is proposed. 1. Introduction 1998; Egbert and Ray, 2000; 2001; Jayne and St. Lau- rent, 2001) have implicated the internal tides as a major Breaking internal waves are the main cause of di- source of mechanical energy for mixing, providing up to apycnal mixing in the ocean’s interior. They may be 1 TW of power to the deep ocean. While some studies generated by wind at the sea surface or by flow over suggest this amount of energy is sufficient for powering the topography of the seafloor. While mean flows and the mixing that closes the meridional overturning cell eddy activity in the deep ocean are characterized by of the ocean’s thermohaline circulation (Webb and Sug- −1 O(1) mm s currents, deep barotropic tidal currents inohara, 2001), other studies suggest that internal tides −1 are O(10) mm s and may be an important source provide only half of the needed energy (Munk and Wun- of internal waves. Recent studies (Munk and Wunsch, 45 46 ST. LAURENT AND NASH sch, 1998). Wind forcing may also supply a significant enhanced turbulence at Cobb Seamount in the north- fraction of the energy input to the internal wavefield east Pacific to a semidiurnal internal tide. They found through the generation of near-inertial swell (Alford, that the largest turbulence levels occurred along a beam 2001,2003a). This highlights the need for studies to of internal tide energy emanating from the seamount examine the relative contributions from each of these rim. Kunze and Toole (1997) describe microstructure sources to internal waves and mixing. observations from Fieberling Seamount in the north- Egbert and Ray (2000, 2001) have identified a number east Pacific. They found the largest mixing levels at of deep ocean regions where barotropic tidal energy is the summit of the seamount where diurnal internal tide likely being transferred to internal tides. These regions energy was trapped. Internal tides are also produced are generally associated with three types of topogra- along the continental margins of the ocean basins, and phy: (i) oceanic islands, (ii) oceanic trenches, and (iii) evidence of internal tide driven mixing at Monterey mid-ocean ridges. Oceanic islands such as Hawaii, and Canyon off California was reported by Lien and Gregg oceanic trenches such as those in the west Pacific Ocean, (2001) and Kunze et al. (2002a). There, enhanced tur- are steep topographic features with typical slopes (s; bulence levels were found along the ray path of a semi- rise over run) of 0.1 to 0.3 (Seibold and Berger, 1996). diurnal internal tide beam extending out a distance of Egbert and Ray (2000, 2001) identify the oceanic islands 4 km away from the topography. Althaus et al. (2003) of Micronesia and Melanesia as sites accounting for over present fine structure observations of internal tides at 100 GW of internal tide production, while Hawaii ac- Mendocino Escarpment. In addition to finding evidence counts for 20 GW. They also identify mid-ocean ridge for strong mixing near the topography, they find en- topography in the Atlantic and Indian Oceans as each hanced mixing at sites where beams of internal tide en- accounting for over 100 GW of internal tide production. ergy refract beneath the sea surface. These topographic features have slopes that vary over Evidence of internal tide driven mixing from the a wide range of spatial scales. truly deep ocean was found during the Brazil Basin Tracer Release Experiment (BBTRE; Polzin et al., a. Observations of internal tides and mixing 1997; Ledwell et al., 2000; St. Laurent et al., 2001). This study was conducted near the Mid-Atlantic Ridge. The contribution of internal tides to oceanic ve- St. Laurent et al. (2001) present vertically integrated locity and temperature records has long been recog- dissipation data that are modulated over the spring- nized. Wunsch (1975) and Hendershott (1981) present neap tidal cycle with a small lag of about a day. reviews of earlier work. Renewed interest in internal Maximum levels of dissipation, vertically integrated to tides came with observations of sea surface elevation by 2000 m above the bottom, reach 3 mW m−2. These the Topex/Poseidon altimeter. These data have been observations span a network of fracture zones west of used to constrain hydrodynamic models of the tides, the Mid-Atlantic Ridge, with topographic relief varying and hence produce accurate estimates of open-ocean by up to 1 km between the crests and floors of abyssal barotropic tides (Egbert et al., 1994; Egbert, 1997). A canyons. The dominant topography of the fracture zone multi-year record of satellite observations is now avail- system has a slope less than 0.1 as the canyons are gen- able, and the semidiurnal tides (M2 and S2)canbe erally 30 to 50 km wide. Elevated turbulent dissipa- dealiased from the record. Ray and Mitchum (1996) tion rates were found along all regions of the fracture used along-track Topex/Poseidon records to examine zone topography, but turbulence levels appeared most the surface manifestation of internal tides generated enhanced over the slopes. Above all classes of topogra- along the Hawaiian Islands. They found that both first phy, turbulence levels decrease to background levels at and second baroclinic modes of the semidiurnal inter- heights above bottom greater than about 1000 m. nal tides were present in the data, and that the sig- Observations of deep, tidally driven mixing have also nal of internal-tide propagation could be tracked up to been made as part of the Hawaii Ocean Mixing Exper- 1000 km away from the Hawaiian Ridge. In a further iment (HOME; Pinkel et al., 2000). Both near- and analysis of the altimetry data, Ray and Mitchum (1997) far-field observational components were conducted, and estimate that 15 GW of semidiurnal internal tide en- fine- and microstructure measurements allowed for es- ergy radiates away from the Hawaiian Ridge in the first timates of tidal energy flux and turbulent dissipation. baroclinic mode. Preliminary HOME observations to be reported here Microstructure observations have provided direct ob- were presented by Kunze et al. (2002b). servations of mixing driven by internal tides at steep Figure 1 shows turbulent dissipation profiles from topography (Lueck and Mudge, 1997; Kunze and Toole, several sites where internal tides are believed to sup- 1997; Lien and Gregg, 2001; Kunze et al., 2002a,b; Al- port mixing. Average profiles from sites of internal tide thaus et al., 2003). Lueck and Mudge (1997) attributed INTERNAL TIDE ENERGY DISSIPATED NEAR TOPOGRAPHY 47 generation include the Mid-Atlantic Ridge of the Brazil Basin (Polzin et al., 1997; Ledwell et al., 2000; St. Lau- rent et al., 2001), and the 3000-m isobath of the Hawai- ian Ridge (Kunze et al., 2002b). The latter was aver- aged from 16 stations spanning roughly ∼1000 km along the Hawaiian Ridge. These stations were occupied over a 3-week period during October 2000 and capture en- ergy flux radiated from both strong and weak sites of internal tide generation along the ridge. Profiles from the Oregon Slope (Moum et al., 2002) and the Virginia Slope (Nash et al., 2003) are also shown, as turbulence at these sites is likely supported by dissipating low mode internal tides. At all sites, the dissipation rates are max- imum along the topography, and decay away from the topography with height. Enhanced turbulence levels are found to extend up to 1000 m from the bottom. The energy flux carried by the internal tide can radi- ate as propagating internal waves, and these waves are subject to a collection of processes that will eventually lead to dissipation.
Details
-
File Typepdf
-
Upload Time-
-
Content LanguagesEnglish
-
Upload UserAnonymous/Not logged-in
-
File Pages14 Page
-
File Size-