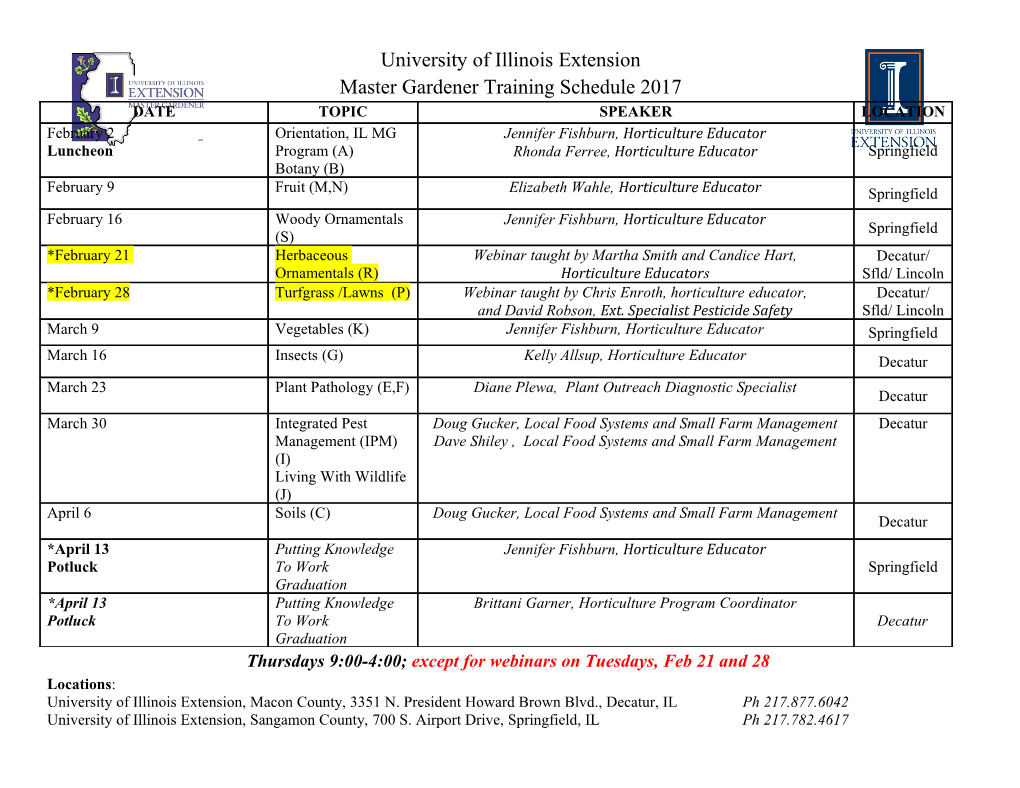
ARTICLE DOI: 10.1038/s41467-018-05269-3 OPEN Unraveling substituent effects on the glass transition temperatures of biorenewable polyesters Xiaopeng Yu 1, Junteng Jia1, Shu Xu 2, Ka Un Lao 1, Maria J. Sanford 1, Ramesh K. Ramakrishnan3, Sergei I. Nazarenko3, Thomas R. Hoye 2, Geoffrey W. Coates 1 & Robert A. DiStasio Jr. 1 Converting biomass-based feedstocks into polymers not only reduces our reliance on fossil fuels, but also furnishes multiple opportunities to design biorenewable polymers with targeted 1234567890():,; properties and functionalities. Here we report a series of high glass transition temperature (Tg up to 184 °C) polyesters derived from sugar-based furan derivatives as well as a joint experimental and theoretical study of substituent effects on their thermal properties. Surprisingly, we find that polymers with moderate steric hindrance exhibit the highest Tg values. Through a detailed Ramachandran-type analysis of the rotational flexibility of the polymer backbone, we find that additional steric hindrance does not necessarily increase chain stiffness in these polyesters. We attribute this interesting structure-property relationship to a complex interplay between methyl- induced steric strain and the concerted rotations along the polymer backbone. We believe that our findings provide key insight into the relationship between structure and thermal properties across a range of synthetic polymers. 1 Department of Chemistry and Chemical Biology, Cornell University, Ithaca, NY 14853, USA. 2 Department of Chemistry, University of Minnesota, Minneapolis, MN 55455, USA. 3 School of Polymers and High Performance Materials, University of Southern Mississippi, Hattiesburg, MS 39402, USA. These authors contributed equally: Xiaopeng Yu, Junteng Jia. Correspondence and requests for materials should be addressed to G.W.C. (email: [email protected]) or to R.A.D.Jr. (email: [email protected]) NATURE COMMUNICATIONS | (2018) 9:2880 | DOI: 10.1038/s41467-018-05269-3 | www.nature.com/naturecommunications 1 ARTICLE NATURE COMMUNICATIONS | DOI: 10.1038/s41467-018-05269-3 s the drive for sustainability continues, petroleum-derived concerted rotations along the polymer chain. In particular, we plastics are steadily being replaced by alternative bio- demonstrate that methyl-induced strain can be leveraged to A 1,2 fl based polymers . In this regard, the conversion of control chain exibility in this class of polyesters by selectively biorenewable feedstocks into aliphatic polyesters has become an destabilizing the relevant minimum-energy conformations across especially desirable path forward as aliphatic polyesters are in the rotational potential energy landscape. On the basis of these general both biodegradable and biocompatible3. During the past findings, we propose several simple and intuitive design principles decade, there has been growing interest in developing synthetic for how methyl substitution can be used to rationalize and tune strategies for copolymerizing epoxides and cyclic anhydrides to Tg trends across a range of synthetic polymers. make such aliphatic polyesters4,5. In addition to providing numerous opportunities for incorporating biorenewable content Results into polymers, these distinct monomer sets also allow us to tune a Monomer synthesis. Acid-catalyzed dehydration of pentoses myriad of polymer properties and functionalities for different (such as xylose) to furfural, and hexoses (such as glucose and applications. For example, we recently found that bulky terpene- fructose) to hydroxymethylfurfural (HMF), represent promising based anhydrides can be employed to make aliphatic polyesters – pathways for the utilization of carbohydrates (Fig. 1a)12 14. with glass transition temperatures (T ) up to 184 °C, thereby g Although many biomass transformations require fermentation, positioning them for use in a number of high-temperature this dehydration protocol proceeds without the use of enzymes, applications6,7. Inspired by this finding, we decided to explore allowing it to be competitive in both cost and productivity13,14.As even more abundant biorenewable feedstocks as building blocks a result, the transformation of furfural and HMF into other value- for high-T materials. g added products and commodities has been the subject of extensive Polymers with high T values often have rigid backbones, which g study13,14. For example, the hydrogenation of furfural and HMF inhibit rotational flexibility in these polymer chains8,9. Hence, one yields 2-methylfuran and 2,5-dimethylfuran, respectively, both of of the most effective ways to increase chain rigidity is by the – which are regarded as potential biofuels (Fig. 1a)12 14. introduction of non-flexible ring structures into the polymer In order to incorporate these sugar-derived biorenewable backbone, thereby hindering (or even eliminating in some cases) feedstocks into polymers, we investigated the Diels-Alder (D-A) rotational flexibility8. As such, aromatic and aliphatic rings are reactions of a number of furan derivatives with maleic anhydride. often found as components of high-T polymers such as aromatic g As previously observed with furfural and maleimide, both furfural polycarbonates, polynorbornenes, and polyimides8. Another and HMF also do not readily undergo a D-A reaction with maleic common way to increase T is by introducing substituents along/ g anhydride, and we attribute this low reactivity to the presence of near the polymer backbone to hinder main-chain rotations the electron-withdrawing aldehyde group and associated higher through steric interactions8,10. Notable examples of such polymers resonance stabilization in these compounds15. In contrast, 2- include poly(α-methylstyrene), poly(2-methylstyrene), and poly methylfuran and 2,5-dimethylfuran readily react with maleic (2,6-dimethylstyrene), all of which are characterized by T values g anhydride to yield thermally labile unsaturated D-A adducts, that are significantly higher than their less substituted analogs11. which can then be hydrogenated or dehydrated to make tricyclic Following these design principles, we report herein several or phthalic anhydrides, respectively, with a targeted number of biorenewable polyesters with ring structures derived from furan, – methyl substituents (Fig. 1b)16 18. 2-methylfuran, and 2,5-dimethylfuran, all of which can be readily synthesized from pentoses and hexoses12–14. In doing so, we observe a quite unexpected structure-property relationship in this Polymer synthesis and characterization. We then systematically class of polymers, namely that the introduction of methyl sub- investigated the copolymerization of these anhydrides using stituents along the polymer backbone does not always increase Tg propylene oxide (PO), 1-butene oxide (BO), and cyclohexene and an intermediate number of methyl substituents actually yields oxide (CHO) in the presence of an aluminum catalyst19 (abbre- F polymers with the highest Tg values. By performing a detailed viated as [ Salph]AlCl) and bis(triphenylphosphine)iminium theoretical analysis of the conformational flexibility in these chloride ([PPN]Cl), as shown in Fig. 2a (see Methods section). polyesters, we provide key chemical and physical insight into the Their unsubstituted analogs (1a, 1d) were also included in this critical role played by methyl-induced steric strain in governing study for comparative purposes, and it is worth noting that the ab O 2 O OH HO O R1 R HO OH + HO O O O HO OH HO OH R1, R2 = H, Me D-xylose D-fructose + + H –H2O H –H2O R1 R2 O O O O O OH O O O Furfural Hydroxymethylfurfural (HMF) + H2, Pd/C H , –H2O cat., H2 –H2O cat., H2 –H2O 1 2 1 2 R O R R R Me O Me O Me O O O O O O 2-methylfuran 2,5-dimethylfuran Fig. 1 Monomer synthesis. a Catalytic conversion of D-xylose and D-fructose into 2-methylfuran and 2,5-dimethylfuran, respectively. b Transformation of substituted furan derivatives into tricyclic (left) and phthalic (right) anhydride monomers 2 NATURE COMMUNICATIONS | (2018) 9:2880 | DOI: 10.1038/s41467-018-05269-3 | www.nature.com/naturecommunications NATURE COMMUNICATIONS | DOI: 10.1038/s41467-018-05269-3 ARTICLE a N N R1 R2 Al F O O F O O Cl O 3 R1 R2 tBu tBu R + O O [PPN]CI, 60 °C O R4 O O R3 R4 n O O Me O Me O Me Me PO O O O O O O O O O O 1a 1b 1c Et BO O Me Me Me O O O O O O O O O 1d 1e 1f CHO b Epoxide comonomer 200 184 PO 180 BO 168 163 CHO 160 156 C) 138 ° ( 140 127 g T 120 120 100 104 100 93 91 85 Copolymer 74 78 78 80 70 63 60 48 40 1a 1b 1c 1d 1e 1f Anhydride comonomer Fig. 2 Polymer synthesis and thermal properties. a Cyclic anhydride and epoxide comonomers investigated in this work. b Observed Tg values for copolymers made from the PO, BO, and CHO epoxides and the tricyclic (1a–1c) or phthalic (1d–1f) anhydride comonomers copolymerization of 1d with various epoxides has been exten- Due to their bulky tricyclic nature, polymers made from 1a–1c 20–24 sively reported in the literature . Under appropriate ratios of are generally characterized by higher Tg values than those made monomer to catalyst, 18 polyesters (Fig. 2) were prepared with from 1d–1f with the same number of methyl substituents well-controlled molecular weights (Mn ~20 kDa for PO and BO (Fig. 2b). When the epoxide was varied, we observed that the copolymers, Mn ~10 kDa for CHO copolymers) and narrow BO-based polymers have lower Tg values than the PO-based dispersities (Supplementary Tables 1–2). No evidence of ether polymers and attribute this to the fact that longer alkyl groups linkage formation was observed by NMR spectroscopy (spectra of often hinder polymer chains from densely packing25,26. Further- – polymers are provided in Supplementary Figures 6 23). Differ- more, the CHO-based polymers have substantially higher Tg ential Scanning Calorimetry (DSC) was used to measure the Tg values relative to both the PO- and BO-based analogs due to the values of all polymers (see Methods section), which are shown in decreased chain flexibility imposed by the cyclohexyl ring8.
Details
-
File Typepdf
-
Upload Time-
-
Content LanguagesEnglish
-
Upload UserAnonymous/Not logged-in
-
File Pages9 Page
-
File Size-