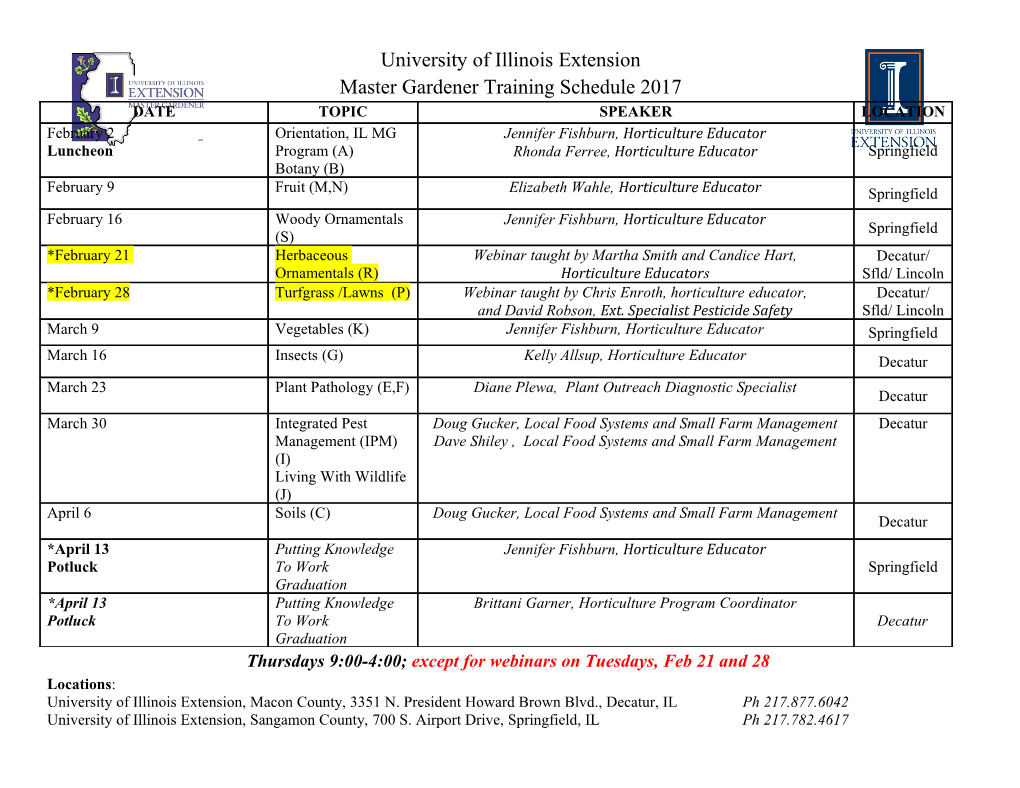
CHAPTER SUBGLACIAL PROCESSES AND SEDIMENTS 5 J. Menzies1, Jaap J.M. van der Meer2 and W.W. Shilts3 1Brock University, St. Catharines, ON, Canada, 2Queen Mary, University of London, London, United Kingdom, 3Illinois State Geological Survey, University of Illinois, Champaign-Urbana, IL, United States 5.1 INTRODUCTION Subglacial processes and sediments, in terms of thickness and areal extent, dominate glaciated con- tinents and ocean basins. Subglacial erosional and depositional processes are complex. Incomplete knowledge of the subglacial environment and its processes and landforms stems from the largely inaccessible environment beneath ice masses and the limited modern analogues to Pleistocene and pre-Pleistocene subglacial environments. This chapter is not exhaustive in the use of older litera- ture. For extended reviews of previous literature, the reader is directed, for example, to Menzies and Shilts (2002), Evans et al. (2006), Benn and Evans (2010) and Schomacker et al. (2010a). Subglacial sediments are of paramount importance to society. The topographic and volumetric abundance of subglacial sediments in glaciated countries cannot be understated. Large areas of all continents where glaciation has occurred, since the earliest of geological times, are covered by these sediments (see chapters: Precambrian Glacial Deposits: Their Origin, Tectonic Setting, and Key Role in Earth Evolution; The Early Palaeozoic Glacial Deposits of Gondwana: Overview, Chronology, and Controversies). The foundations of roads, railroads, aircraft runways, the waste disposal sites for sew- age, toxic waste sites, the utilization of agricultural land (see chapter: Glacioaeolian Processes, Sediments, and Landforms), the distribution and movement of groundwater in aquifers, the utilization of glacial sediments in drift prospecting (see chapter: Drift Prospecting in Glaciated Terrain) and use of glacially derived construction materials in those areas last glaciated in the Pleistocene, are all dependent on subglacial sediments, their structural architecture, grain size and stratigraphic complex- ity. As our increasing awareness of environmental hazards and rational land use develops in the 21st century, the demand for expert and applied knowledge of glacial sediments and environments will rise, with a prime place given to knowledge of the subglacial environment (De Mulder and Hageman, 1989; Coates, 1991; Knight, 2008; Paulen and McMartin, 2009). Historically, major population cen- tres developed in areas that were glaciated because of the rich soils that promoted agriculture provid- ing sustenance for large populations. Perhaps no other glacial environment impacts on the daily lives of millions of people to such a high degree as does the subglacial (Box 5.1). The subglacial environment can be viewed as containing a series of sediment packages that each have boundary interfaces that are sandwiched between an upper ice mass and an underlying Past Glacial Environments. DOI: http://dx.doi.org/10.1016/B978-0-08-100524-8.00004-X © 2018 Elsevier Ltd. All rights reserved. 105 106 CHAPTER 5 SUBGLACIAL PROCESSES AND SEDIMENTS BOX 5.1 DEFINITION Where the subglacial environment ceases and proglacial, submarginal and/or subaqueous glacial environments commence remains, at times, debatable. The subglacial environment is that glacial subsystem directly underlying an ice mass in close contact with the overlying ice, including those cavities and channels beneath the ice that are not directly influenced by subaerial processes. On land, the subglacial environment may continue for some distance beyond the apparent surface ice margin since buried active ice may underlie debris that has been deposited either from supraglacial sources or from various meltwater streams exiting the ice (Fig. 5.1A, B)(Bernard et al., 2014; Banwell et al., 2016; Hiester et al., 2016). Likewise, on entering a body of water an ice mass may be sufficiently thick or the water sufficiently shallow to permit ice to remain in contact with the bed, the basal environment thereby remaining subglacial. Where ice begins to rise off its bed due to buoyancy from lake or oceanic waters, the subglacial environment can be said to cease down-ice of this dynamic hinge point, the grounding-line (Fig. 5.1C). Down-ice from a grounding-line an extensive proglacial subaqueous proximal environment may exist. Such a proximal subaquatic zone may become transiently subglacial for short periods of time and at different places on the bed (see chapters: Modern Glaciomarine Environments and Sediments; Glaciovolcanism: A 21st Century Proxy for Palaeo-Ice). bed (composed of bedrock or underlying sediments) where a complicated set of processes interact altering the morphological, thermal and rheological state of the sandwiched sediments, evolving into several and, at times, a single interface junction (cf. Smith et al., 2015) (see Fig. 5.8). The nature of this interface controls glacial erosion, transportation and deposition processes. The key to subglacial bed types and associated forms lies in understanding the mechanics of this complex set of interfaces. These boundary zones migrate across the landscape with every advance and retreat of the ice masses. Therefore, all terrains covered by glaciers have been affected and altered by the passage of this boundary zone. Subglacial depositional and erosional phenomena are often destroyed, radically altered or obscured by later processes associated with proglacial environments following gradual ice retreat or by more complex subglacial overriding and subsequent ice retreat, or by subaerial diagenesis. The transport of sediments within the glacial system requires an understanding of the sediment delivery systems (SDS) within supraglacial, proglacial and subglacial subenvironments. Glacial deposits and landforms are end products of these sediment systems (Evans, 2003). Sediment deliv- ery occurs as a function of ice dynamics, the type of ice mass, basement and subjacent geology and sedimentology, the temporal and spatial variability of sediment discharge (flux), associated hydro- logical regimens and topography (Menzies et al., 2016; Spagnolo et al., 2016). Fig. 5.1 illustrates the many and complex pathways of these transport systems. Individual local sediment delivery systems depend on ice internal and basal thermal conditions, whether polar or temperate (Menzies and Shilts, 2002; Frederick et al., 2016; MacGregor et al., 2016). Under polar frozen bed conditions, it was once thought that little or no transport of sediment occurred other than in the supraglacial system. However, evidence now shows that even under polar or subpolar bed conditions, some limited transportation occurs as part of a slow deforming bed (Shreve, 1984; Echelmeyer and Zhongxiang, 1987; Hallet et al., 1996; Alley et al., 1997; Lloyd Davies et al., 2009)(Table 5.1). In contrast to frozen bed conditions, large quantities of sediment are transported in all the sediment subsystems under temperate wet bed conditions (Vorren and Laberg, 1997; Kirkbride, 2002; Ottesen et al., 2005, 2016; O’Brien et al. 2007; Benn and Evans, 2010; Schomacker et al., 2010b)(Fig. 5.1). FIGURE 5.1 Sediment delivery systems (SDS) within glacial environments: (A) SDS within valley glacial systems, (B) SDS within marginal ice sheet systems, and (C) SDS within subaqueous glacial environments. (A) Modified from Boulton, G.S., Eyles, N., 1979. Sedimentation by valley glaciers; a model and genetic classification. In: Schlu¨chter, C. (Ed.), Moraines and Varves: Origin/Genesis/Classification. A.A. Balkema, Rotterdam, pp. 11À23. 108 CHAPTER 5 SUBGLACIAL PROCESSES AND SEDIMENTS Table 5.1 Subglacial Interface Types As ice conditions fluctuate within an ice mass over diurnal, seasonal and annual cycles, there changes occur in the volume and rate of sediment transfer. Daily, as discharge of meltwater increases as a function of solar radiation, transport of both bedload and suspended sediment increases within the supraglacial, englacial and proglacial subenvironments. There may be a slight daily increase in subglacial and englacial transport rates as meltwater penetrates a thin ice mass, such as a cirque or valley glacier or the marginal edge of an ice sheet. In general, however, sediment transport in subgla- cial systems is likely to remain relatively steady, even over an annual cycle (Truffer et al., 2000; Habermann et al., 2013). Seasonal changes in sediment transport are influenced by local weather con- ditions. When freezing temperatures prevail, supraglacial and subsequently englacial meltwater trans- port ceases. At the same time, subglacial transport is likely to be restricted, there being little or no additional surface meltwater but frictional heating and geothermal effects maintain some level of sub- glacial meltwater transport under temperate basal conditions (Ottesen et al., 2005; Nyga˚rd et al., 2007; Quincey and Luckman, 2009; Dowdeswell and Ottesen, 2016). It is evident that sediment transported by ice masses reflects the underlying and surrounding lat- eral (in the case of valley glaciers) and up-ice bedrock geology (see chapter: Application of Till Mineralogy and Geochemistry to Mineral Exploration). Although ice masses may transport sedi- ment extremely long distances of hundreds of kilometres (Shilts, 1977, 1984, 1993; Meer and Wicander 1992; McClenaghan and Peter, 2016). Much of the sediment load in the subglacial sys- tem, is derived from little more than a few kilometres up-ice, from as little as 10À15 km (Dreimanis and Vagners,
Details
-
File Typepdf
-
Upload Time-
-
Content LanguagesEnglish
-
Upload UserAnonymous/Not logged-in
-
File Pages54 Page
-
File Size-