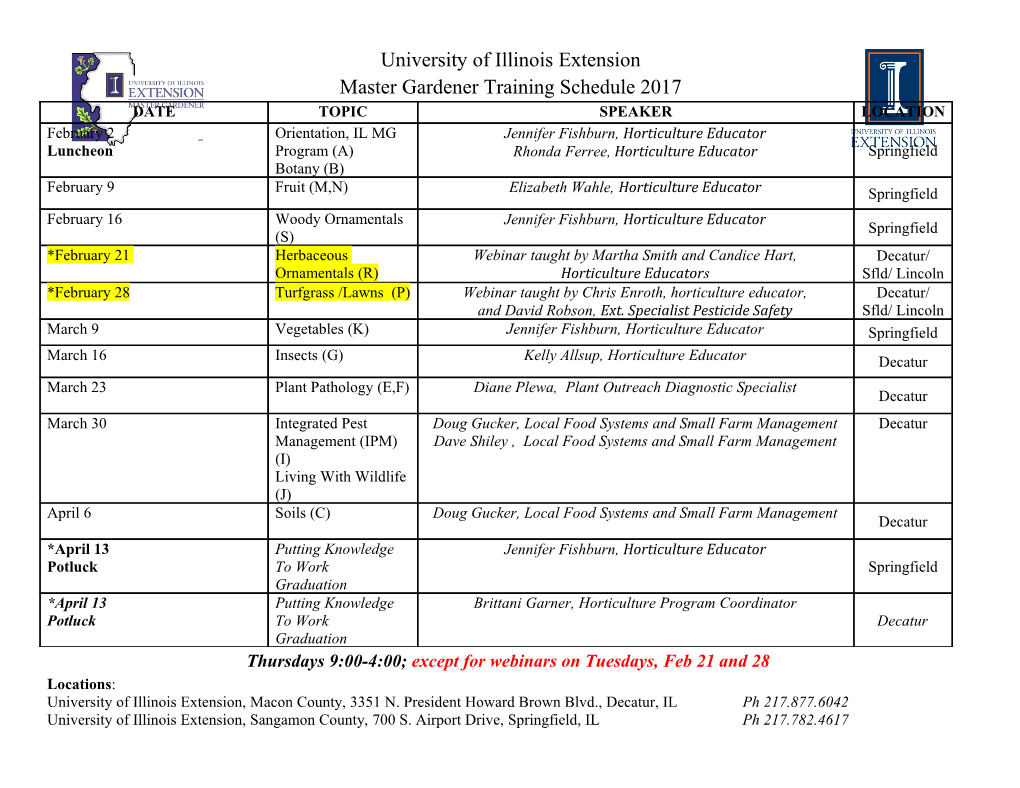
High Power Ultrafast Yb:fiber Laser A Thesis presented by Xinlong Li to The Graduate School in Partial Fulfillment of the Requirements for the Degree of Master of Science in Instrumentation (Physics) Stony Brook University August 2015 Stony Brook University The Graduate School Xinlong Li We, the thesis committe for the above candidate for the Master of Science degree, hereby recommend acceptance of this thesis Thomas K.Allison - Thesis Advisor Assistant Professor, Department of Physics and Astronomy, Department of Chemistry Eden Figueroa - Committee Member Assistant Professor, Department of Physics and Astronomy Matthew Dawber - Committee Member Associate Professor, Department of Physics and Astronomy Meigan C. Aronson - Committee Member Professor, Department of Physics and Astronomy This thesis is accepted by the Graduate School Charles Taber Dean of the Graduate School ii Abstract of the Thesis High Power Ultrafast Yb:fiber Laser by Xinlong Li Master of Science in Instrumentation (Physics) Stony Brook University 2015 High power ultrafast laser pulses have broad applications in many fields such as mi- cromachining, real time imaging of ultrafast process, and frequency-comb-based precision spectroscopy, enabling large numbers of breakthroughs in science and technology. They even open the door to the attosecond (10−18 s) world via high harmonic generation thus gaining the insight into a wide range of electron dynamics. In this thesis, I present a linearly amplified Yb:fiber laser with 55 W average output power and 150 fs pulse duration. This laser is used for cavity-enhanced high harmonic generation to produce high-repetition-rate (78 MHz) extreme ultraviolet (XUV) femtosecond pulses. iii Contents List of Figures v List of Tables viii Acknowledgement ix 1 Introduction 1 1.1 Mode-Locking . 1 1.2 Frequency Comb . 3 1.3 Pulse Propagation . 4 1.4 High Harmonic Generation . 6 1.5 Fiber Laser . 9 1.6 Nonlinear Effect . 11 2 High Power Yb:Fiber Comb 16 2.1 Oscillator . 16 2.2 Comb Tooth Linewidth . 18 2.3 Stretcher . 21 2.4 Optical Amplifier . 22 2.5 Compressor . 25 2.6 FROG Measurement and Pulse Duration . 25 2.7 Beam Mode . 28 2.8 Relative Intensity Noise . 29 2.9 Interlock . 30 2.10 LabVIEW Control . 32 2.11 Mechanical Design and Cooling system . 32 3 Conclusion 35 Bibliography 38 Appendix 43 A. LabView code . 43 iv List of Figures 1.1 Spectrum consists of discrete lines with equal spacing. 1 1.2 (a) 10 frequency modes without model-locking. (b) Mode-locking with 2, 5, 10 frequency modes, respectively. 2 1.3 Schematic of a conventional pulse laser. 2 1.4 (a) The spectrum of frequency comb. Each comb tooth is equally spaced and has a very narrow bandwidth of usually kHz level.. (b) Pulse train in the time domain. Pulses are equally spaced by the time T = 1=f. The phase difference between carrier and envelope is shifted by ∆Φ between adjacent pulses. 4 1.5 Schematic of compressor (top view). Light with longer wavelength will travel longer distance than shorter wavelength. G1 and G2 are gratings. G: normal distance between gratings. Roof reflector changes the beam hight so we can sort out the output beam of the compressor. Detailed information of parts is in table 3. 5 1.6 Refractive index distribution of dressed cladding fiber [1] . 6 1.7 Three step model for HHG. 6 1.8 Conventional HHG schematic. Infrared femtosecond laser pulses are focused into a gas to achieve intensities in the strong field regime. A metallic filter blocks the fundamental driving pulses . 7 1.9 (a) Attosecond photon burst at each half wave cycle. (b) Single attosecond burst each pulse. (c) Spectrum of (a). !0 is the central frequency of funda- mental driving laser. !c is the cut off frequency where the yield of harmonics start to drop. (d) Spectrum of (b). 8 1.10 Setup of intracavity HHG chamber. Fundamental driving pulses are focused to argon gas to generate XUV pulses. The XUV pulses are coupled out by using a crystal at Brewster angle. 9 1.11 Illustration of phase locking (frequency domain). 9 1.12 Schematic of a high-power, double-clad fibre amplifier. 10 1.13 (a) Fiber end image of NKT DC-200/40-PZ-Yb. (b) Fiber end image of NKT aeroGAIN-ROD-PM85. The little circle structures are air holes. 10 1.14 Absorption and emission cross section of Ytterbium [45]. 11 1.15 MATLAB simulation of gain narrowing (code from Donald Willcox). 12 1.16 MATLAB simulation of SPM of pulse after propagation along a piece of fiber (dispersion in the fiber is ignored). New frequency component can be observed. 13 1.17 Simulation by MATLAB (code from Donald Willcox). The upper figures show the power distribution along the fiber. The lower figures show the accumulated B-integral along the fiber. CTP stands for counter propagating between pump laser and signal laser. COP stands for co-propagating. 14 2.1 The main parts of the high power IR laser . 16 2.2 Scheme of oscillator. Detailed information of parts is in table 1. 17 2.3 Spectrum of oscillator with two different WDMs. 19 2.4 Spectrum teeth beat with Nd:YAG laser. Rep rate stands for repetition rate. Four of the beat note signals are marked (n = 0 and n = 1). 19 v 2.5 Repetition rate signal and beat note signal measured by spectrum analyzer. Signal at 156 MHz is the harmonic of the repetition rate signal. The beat note signals with n = 1 is shown. 20 2.6 Beat signal measured with different RBW . 20 2.7 Scheme of stretcher (Figure from OFS website) . 21 2.8 Schematic of optical amplifier. The 5 m crystal fiber is the pre-amplifier and the 0.8 m crystal fiber is the rod-type power amplifier. The detailed information of the parts (L1, L2, D1, etc) can be found in table 2. L stands for lens. D stands for dichroic filter. I stands for isolator. M stands for plane mirror. HWP stands for half wave plate. BD stands for beam damp. 22 2.9 (a) Amplification Curve of Pre-amplifier. (b) Amplification Curve of power amplifier before compression and after compression. 24 2.10 (a) Power amplifier spectrum of its seed light, 25 W amplified light and 70 W amplified light. The spectrum of oscillator is also shown. (b) The reflectivity of the dichroic mirrors D1 and D2 (D4), which show cutoff at ∼1010 nm and ∼1030 nm, respectively. This is single measurement without averaging. The data for D2 has been squared because the output of pre-amplifier will be reflected twice (D2 and D4) before going into the power amplifier. 24 2.11 A schematic of FROG measurement. BS is Beamsplitter. M1 and M3 are plane mirrors. M2 is a focusing mirror. The stage can control the delay time of split pulses . 26 2.12 Raw data of FROG, measured SHG intensity at different delay and different wavelength . 26 2.13 (a) Reconstructed temporal pulse shape at low power and high power. Du- ration is 150 fs. The Fourier transform limited pulse (120 fs) is obtained by Fourier transform from measured spectrum (figure 2.10a). (b) Reconstructed spectrum and spectral phase at 55 W. 27 2.14 (a) Temporal pulse shape when third order phase dominates. (b) Spectrum and spectral phase when third order phase dominates. 27 2.15 (a) Beam mode at low output power (2 W) (b) Beam mode at high output power (55 W). Unit in µm............................ 28 2.16 Measured RIN of oscillator, pre-amplifier and power-amplifier. Each curve is combined by three measurements from left to right: 6.25 kHz span using FFT spectrum analyzer, 100 kHz span using FFT spectrum analyzer, 2 MHz span using Rigol spectrum analyzer, . 29 2.17 Circuit diagram of the interlock (Designed by Melanie Raber and Yuning Chen) 31 2.18 Laser Control Program by LabVIEW . 32 2.19 Beam damp. BD1 in figure 2.8. 33 2.20 Beam damp. BD2 in figure 2.8. 33 2.21 Fully water cooling for rod fiber. Water flows in from port 1 and flows our from port 2. 34 3.1 Schematic of cavity HHG, monochromator and surface experiment. 35 3.2 Photo of high power ultrafast laser. Pre-amplifier (green fiber) and power amplifier (straight rod) are shown. 36 3.3 Photo of HHG chamber and monochromator chamber. 36 vi 3.4 Photo of surface sciences chamber. 37 A1 LabView code part 1 . 43 A2 LabView code part 2 . 43 A3 LabView code part 3 . 44 A4 LabView code part 4 . 44 A5 LabView code part 5 . 45 A6 LabView code part 6 . 45 vii List of Tables 1 Detailed information of parts in the oscillator (figure 2.2). 18 2 Detailed information of parts in the optical amplifier (figure 2.8). 23 3 Detailed information of parts in the compressor (figure 1.5). GD stands for groove density. 25 4 Dispersion parts in the laser. Normal dispersion: φ2 < 0, φ3 < 0. Anomalous dispersion: φ2 > 0, φ3 > 0. FS stands for fused silica. TGG stands for Terbium Gallium Garnet crystal. 25 viii Acknowledgement This work owes much to the people who provided me help and opportunity. I want to thank my advisor professor Thomas Allison who allowed me to join his research group and choose this project for me. I have learned a lot of knowledge and skills in this project. I want to thank Christopher Corder for the instruction and cooperation during this project. I also want to thank Peng Zhao who worked with me. Thanks to Melanie Reber and Yuning Chen for their initial work and continuous help in this project.
Details
-
File Typepdf
-
Upload Time-
-
Content LanguagesEnglish
-
Upload UserAnonymous/Not logged-in
-
File Pages54 Page
-
File Size-