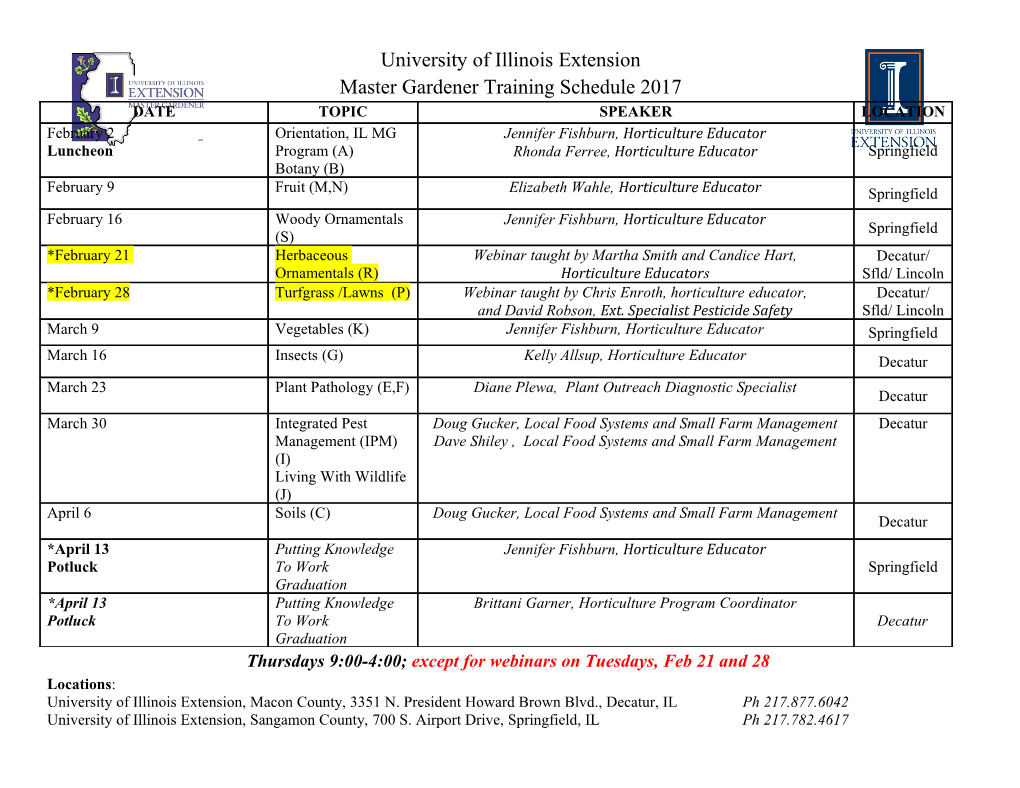
Attosecond two-photon interferometry for doubly excited states of helium J. Feist,1, * S. Nagele,2, y C. Ticknor,3 B. I. Schneider,4 L. A. Collins,3 and J. Burgdörfer2 1ITAMP, Harvard-Smithsonian Center for Astrophysics, Cambridge, Massachusetts 02138, USA 2Institute for Theoretical Physics, Vienna University of Technology, 1040 Vienna, Austria, EU 3Theoretical Division, Los Alamos National Laboratory, Los Alamos, New Mexico 87545, USA 4Office of Cyberinfrastructure/Physics Division, National Science Foundation, Arlington, Virginia 22230, USA (Dated: November 20, 2018) We show that the correlation dynamics in coherently excited doubly excited resonances of helium can be followed in real time by two-photon interferometry. This approach promises to map the evolution of the two-electron wave packet onto experimentally easily accessible non-coincident single electron spectra. We analyze the interferometric signal in terms of a semi-analytical model which is validated by a numerical solution of the time-dependent two-electron Schrödinger equation in its full dimensionality. PACS numbers: 32.80.Fb, 32.80.Rm, 42.50.Hz Advances in optical technologies and laser sources in excitation by charged particles as pump and the velocity the past decade led to the production of extreme ultra- of post-collisional energy shifts as probe. From the veloc- violet (XUV) light pulses as short as 80 attoseconds (1 ity dependence of the angular differential autoionization attosecond = 10−18 seconds) [1–3]. Thus, the direct ex- spectra (“PCI effects”) the time evolution of collective ploration of the electronic dynamics in atoms, molecules two-electron variables such as the dipole, ~r1 + ~r2 (or h i and solids in the time domain came into reach. This Runge-Lenz vectors ~a1 +~a2 ), or the vibronic motion of h i advance initiated a whole new field, attosecond physics, the interelectronic angle ~a1 ~a2 could be identified and several pioneering experiments exploiting the novel [11]. For a XUV-XUV pump∼ h probe· i scenario a few the- technologies have already been performed (see [4–6] and oretical proposals to guide attosecond-pulse experiments references therein). Most measurement protocols ei- have been put forward. Hu and Collins [12] proposed ther realized or proposed rely up to now on an inter- to map out the wavepacket in coherently singly excited play of an attosecond XUV pulse and a few-cycle IR helium created by the pump pulse. They performed ab- pulse with durations 휏IR & 5 fs. Sub-fs time resolu- initio calculations for the double ionization by the probe tion is achieved through the exquisite sub-cycle control pulse as a function of delay time 휏 and showed that the over carrier-envelope phase (CEP) stabilized IR pulses total double ionization signal oscillation directly mirrors −2 with uncertainties as small as Δ' 10 T0 where T0 the radial breathing motion in the singly-excited state is the period of the IR oscillation.≈ However, the di- manifold. This scenario requires, however, a two-color rect analogue to femtosecond pump-probe spectroscopy XUV-XUV pump-probe sequence. Morishita et al. [13] in chemistry on the attosecond scale, i.e., excitation of showed, within lowest order perturbation theory, that the an electronic wavepacket by an attosecond pump pulse correlated motion of the two electrons in a wavepacket followed by an attosecond probe pulse to take snapshots among the doubly excited states (DES) of helium can be of the ensuing electronic motion remains to be accom- resolved by an XUV-XUV pump-probe scheme provided plished. One obvious difficulty is that current attosecond that the full six-dimensional two-electron momenta of the XUV pulses based on high-harmonic generation (HHG) ejected electrons are resolved in a kinematically complete had, up to now, insufficient intensity to efficiently realize experiment. multi-photon pump-probe protocols. Very recently, how- In this letter we present a novel single-color XUV-XUV arXiv:1104.3798v1 [quant-ph] 19 Apr 2011 ever, significant increases in HHG efficiency have been interferometric pump-probe protocol that allows to fol- reported [7,8]. Therefore, attosecond XUV-XUV pump- low the correlated two-electron motion in doubly excited probe experiments, which have been dubbed the “holy states in real time by observing only (relatively) easily ac- grail” of attosecond physics [5], will likely be realized in cessible integral and non-coincident experimental observ- the near future opening up a new stage of attosecond ables. To map out the electronic dynamics we exploit the science. interference between three two-photon double ionization This experimental perspective challenges theory to pathways (see Fig. 1) in a fashion which greatly enhances identify observables readily accessible in the experiment the observable signal. that map out non-trivial wavepacket dynamics of corre- We solve for the proposed scenario the time-dependent lated electronic motion. The paradigm system for corre- Schrödinger equation in its full dimensionality, includ- lated electron dynamics in real time are manifolds of co- ing electronic correlations without further approxima- herently excited doubly excited states (i.e., resonances) in tions (see [14]). The numerical parameters were chosen helium. Pioneering experiments [9, 10] utilized collision to ensure convergence. The XUV pulses have a sin2 enve- 2 path α 50 10 40 He++ 2 1 30 [eV] β 2 path DES 20 0.1 + 1 He 10 0 0.01 path γ 0 10 20 30 40 50 1s2 1 [eV] FIG. 2. Two-photon double ionization spectrum in the (휖1; 휖2) plane for a pump-probe sequence of two 1 fs sin2 20 nm pulses FIG. 1. Three-path interferometer for attosecond two-photon with a peak-to-peak delay of 휏 = 1500 as. The white lines de- double ionization probing the coherent dynamics in doubly limit the spectral window without contamination by sequen- excited states (DES). The three paths 훼, 훽, and 훾 are repre- tial contributions. The diagonal oscillations in 휖1 + 휖2 result sented by blue, green, and red arrows, respectively (see text). from the interference between pathways 훼 and 훽 + 훾. The Interference areas ΔE휏: Area 1 (light-green) is delineated complex interference pattern within the “sequential” peaks at (! − I1;! − I2) is primarily due to interference between 훼, 훾, by (quasi-) bound states and is stable under average over 휖1 and the sequential pathway with one photon from each pulse (or 휖2). Area 2 (in light-blue) is delineated by the energy (see text). E = 휖1 + 휖2 of the two-electron continuum state and varies rapidly under variation of 휖1 (or 휖2). gram (Fig. 1) between path 훼 and any other path rapidly lope with total duration of 1 fs, a FWHM of intensity of varies over the Fourier width of the total final energy, 390 as, and a central energy ! of 65:3 eV. All calculations 휖1 + 휖2, in the continuum (along the diagonal in Fig. 2). presented in the following were performed for peak in- Any partial trace over unobserved variables, e.g. the en- tensities of 1012 W=cm2 for rapid numerical convergence. ergy of one electron, will wipe out any interference fringes For the experiment, values close to 1015 W=cm2 would associated with path 훼 and will result in an incoherent be desired. We have explicitly checked that our results and 휏-independent background contribution to the ob- remain valid at such intensities, three-photon processes served electron spectra. and ground state depletion are still negligible. For energies close to (휖1; 휖2) = (! I1;! I2) and its − − The present attosecond two-photon pump-probe se- exchange symmetric partner (! I2;! I1) where I1 and − − 2 + quence (Fig. 1) of DES can be viewed as a three-path I2 are the ionization thresholds of He(1s ) and He (1s), interferometer, with the time delay 휏 between the pulses the additional pathway of sequential two-photon ioniza- corresponding to the “arm length” of the interferometer. tion, creating first He+(1s) by the pump and then He++ Path 훼 corresponds to two-photon double ionization by by the probe (omitted from Fig. 1 for clarity) gives rise the pump pulse which has been the subject of a large to additional rapidly oscillating fringes within the Fourier number of recent investigations (see e.g. [14] and ref- broadened “sequential peaks” (see Fig. 2). They can be erences therein). Path 훾 is its replica induced by the removed by choosing an appropriate spectral window for probe pulse delayed by a time interval 휏 relative to the the one-electron energies (! I2) < 휖 < (! I1) within pump pulse. The intermediate path 훽 represents a proper the “sequential” peaks. Focusing− in the following− on this pump-probe sequence where the first one-photon transi- energy window and integrating over the energy of the sec- tion coherently excites a wavepacket of an ensemble of ond electron leaves us with interference fringes that are 훽 훾 doubly excited states whose time evolution is then probed exclusively determined by the phases, 휑m = (Em E0 )휏. by double ionization by the second photon after the delay The enclosed area (Fig. 1) is delimited by the two− sharp time 휏. Two specific features of this three-path interfer- boundaries of the quasi-bound states of resonances (path- 2 ometer, which displays a complex fringe pattern in the way 훽) and by the ground state He(1s ) with energy E0 (휖1; 휖2) plane of final energies of electron 1 and 2 (Fig. 2), (pathway 훾). Since the DES wavepacket encompasses 훽 are key to resolving the DES wavepacket dynamics. First, several resonances with energies Em (m = 1;:::), the path 훼 represents a “fuzzy” slit. The interference phase resulting interference fringes will display a fast oscilla- ΔE휏 represented by the area enclosed in the E t dia- tion on the attosecond scale given by the average phase − 3 1P e symmetry is accessed, and we use the traditional but 3.2 numerical results imprecise labels 2snp± for brevity.
Details
-
File Typepdf
-
Upload Time-
-
Content LanguagesEnglish
-
Upload UserAnonymous/Not logged-in
-
File Pages5 Page
-
File Size-