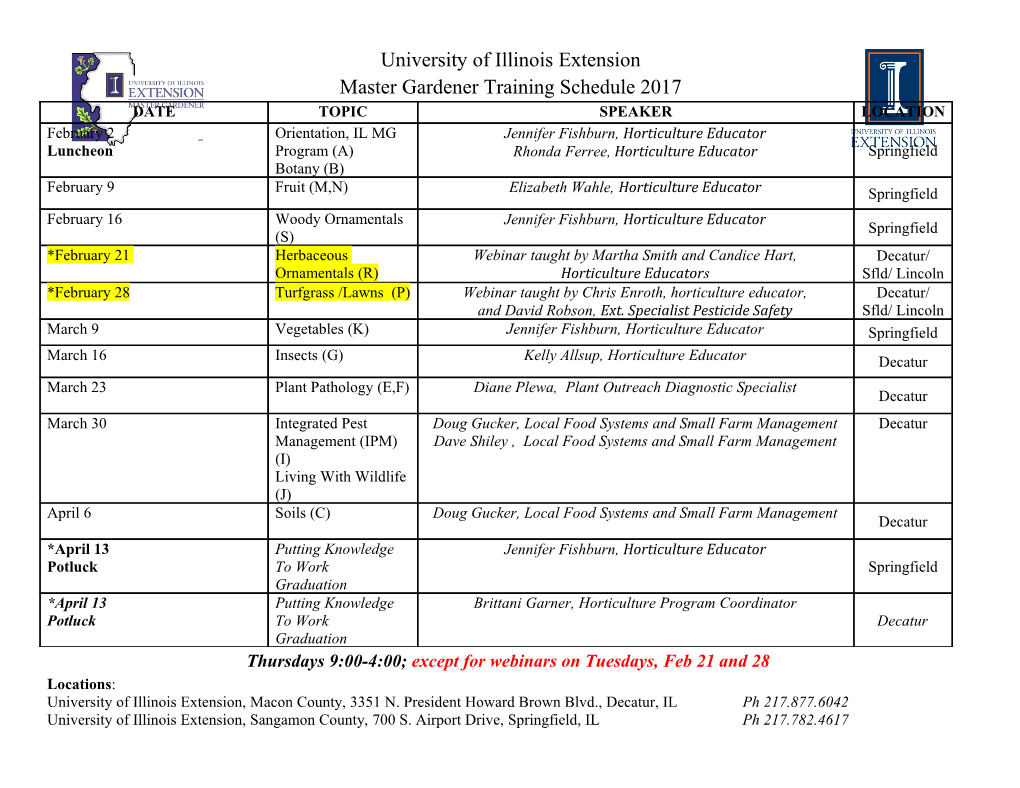
3356 JOURNAL OF THE ATMOSPHERIC SCIENCES VOLUME 72 The Atmospheric Response to Weak Sea Surface Temperature Fronts* NIKLAS SCHNEIDER International Pacific Research Center, and Department of Oceanography, University of Hawai‘i at Manoa, Honolulu, Hawaii BO QIU Department of Oceanography, University of Hawai‘i at Manoa, Honolulu, Hawaii (Manuscript received 17 July 2014, in final form 27 April 2015) ABSTRACT The response of the atmospheric boundary layer to fronts of sea surface temperature (SST) is characterized by correlations between wind stress divergence and the downwind component of the SST gradient and between the wind stress curl and the crosswind component of the SST gradient. The associated regression (or coupling) coefficients for the wind stress divergence are consistently larger than those for the wind stress curl. To explore the underlying physics, the authors introduce a linearized model of the atmospheric boundary layer response to SST-induced modulations of boundary layer hydrostatic pressure and vertical mixing in the presence of ad- vection by a background Ekman spiral. Model solutions are a strong function of the SST scale and background advection and recover observed characteristics. The coupling coefficients for wind stress divergence and curl are governed by distinct physics. Wind stress divergence results from either large-scale winds crossing the front or from a thermally direct, cross-frontal circulation. Wind stress curl, expected to be largest when winds are parallel to SST fronts, is reduced through geostrophic spindown and thereby yields weaker coupling coefficients. 1. Introduction stress divergence and curl are proportional to the Satellite-borne observations of the atmospheric re- downwind and crosswind gradients of SST, respectively sponse to fronts of sea surface temperature (SST) have (O’Neill et al. 2003; Chelton and Xie 2010; Song et al. revolutionized the understanding of midlatitude air–sea 2009). The associated regression coefficients (i.e., cou- interaction (Xie 2004; Small et al. 2008). While the tra- pling coefficients) vary seasonally and regionally, but ditional, large-scale view holds that the ocean primarily the coupling coefficients between divergence and responds to forcing by the atmosphere, the ocean me- downwind SST gradients are consistently larger than soscale shows a ubiquitous imprint of SST fronts on the those between wind stress curl and crosswind SST gra- atmospheric boundary layer (Chelton and Xie 2010; Xie dients in observations (Chelton and Xie 2010) and in 2004). For scales shorter than about 1000 km, wind high-resolution numerical models (Seo et al. 2007; Song speeds are proportional to SST perturbations, and wind et al. 2009; Bryan et al. 2010). Kinematically, this results from gradients of the frontally induced surface stress direction that diminish the wind stress curl but enhance Denotes Open Access content. the wind stress divergence (O’Neill et al. 2010a). Here, we seek to dynamically explain these observations using a linearized model for the atmospheric boundary layer that * International Pacific Research Center Publication Number 1119 and School of Ocean and Earth Science and Technology includes advection by background Ekman winds, fron- Publication Number 9423. tally induced air–sea fluxes of heat, and their impact on the momentum budget. In the process, we provide a unified framework for all processes put forth in the con- Corresponding author address: N. Schneider, International Pa- cific Research Center, University of Hawai‘i at Manoa, 1680 East text of frontal air–sea interaction, cast frontal air–sea West Road, Honolulu, HI 96822. interaction as a classical Rossby adjustment problem, and E-mail: [email protected] explore its scale and parameter dependence. DOI: 10.1175/JAS-D-14-0212.1 Ó 2015 American Meteorological Society SEPTEMBER 2015 S C H N E I D E R A N D Q I U 3357 Two mechanisms are invoked to explain the response baroclinic and back pressures. The role of advection that of boundary layer winds and surface stresses to fronts of leads to imbalances of boundary layer temperature and SST: adjustments of vertical mixing and of baroclinic SST and that affects the boundary layer momentum pressure gradients. Modulation of air–sea temperature budget is not considered. fluxes affects vertical mixing between winds near surface Recent modeling studies (Kilpatrick 2013; Kilpatrick and aloft and accelerate (decelerate) surface winds et al. 2014) that build upon the two-dimensional simu- downstream of a cold-to-warm (warm-to-cold) front lations of frontal air–sea interaction (Wai and Stage (Wallace et al. 1989; Hayes et al. 1989). The increase in 1989; Spall 2007) show the dynamics of frontal in- vertical mixing and associated deepening of the teraction to be a strong function of the large-scale wind boundary layer may be limited to the wake of the non- magnitude and direction relative to the front. For strong equilibrated difference of boundary layer and sea sur- cross-frontal winds, the effective time scale of an air face temperatures, or it may extend farther downstream column crossing the front is shorter than an inertial if the boundary layer depth is permanently altered period. Resulting boundary layer transport divergences (Samelson et al. 2006). Support for this process comes excite vertically propagating gravity waves in the free from observations of higher surface winds (Sweet et al. troposphere (Kilpatrick et al. 2014). In contrast, for 1981) and deep boundary layers on the warm side of SST alongfront winds, the effective time scale experienced fronts (Businger and Shaw 1984; Wai and Stage 1989). by an air column that crosses the front is longer than an Air–sea temperature fluxes downstream of an SST front inertial period so that a surface trapped response results also imprint the oceanic conditions on the hydrostatic, consistent with a spindown of the lower troposphere baroclinic pressure in the boundary layer (Lindzen and induced by the frontally induced secondary circulation Nigam 1987). Evidence for this pressure effect comes (Kilpatrick 2013). from the covariations of divergences of surface winds and In this manuscript, we explore the hypothesis that surface wind stress with the Laplacian of surface tem- spindown is responsible for the consistently smaller perature and sea level pressure (Shimada and Minobe magnitude of the coupling coefficients for the wind 2011; Tokinaga et al. 2009; Lambaerts et al. 2013). stress curl than for the wind stress divergence. To this The distinct responses of the wind stress divergence end, we adopt a reduced-gravity model for the atmo- and curl emerge from the response of the atmospheric spheric boundary layer (Lindzen and Nigam 1987; boundary layer momentum, heat, and mass balances to Battisti et al. 1999) to include advection by a prescribed, the accelerations induced by vertical mixing and pres- uniform, geostrophic wind and forcing by an arbitrary sure gradient mechanisms. In the absence of horizontal SST distribution (sections 2 and 3). We explore the re- advection, Ekman pumping due to the divergence of the sponses of the model vis-à-vis observed characteristics frontally induced Ekman transports displaces the strati- (section 4), and show the distinct dynamics governing fication outside of the boundary layer and generates a the wind stress divergence and curl and their coupling back pressure in the boundary layer (Lindzen and Nigam coefficients (section 5). Parameter sensitivity of the re- 1987; Battisti et al. 1999) that spins down (Greenspan and sults and a comparison with observations are presented Howard 1963; Holton 1965a,b; Pedlosky 1967)thewind (section 6), followed by conclusions (section 7). stress curl.1 Atmospheric observations show the back pressure, in that frontal adjustments extend beyond the 2. Reduced-gravity model boundary layer and partially offset the surface pressure gradients induced by mesoscale SST (Hashizume et al. We employ a minimal model that includes the frontal 2002). A series of numerical investigations on the re- physics outlined above and simplifies the vertical structure sponse of the free troposphere to SST fronts relies on of the lower atmosphere as an active layer adjacent to the this frontally induced Ekman pumping to couple the surface separated by a sharp inversion from the resting boundary layer with the atmosphere aloft (Feliks et al. troposphere aloft (Battisti et al. 1999). The full, non- 2004, 2007, 2011). In these studies, the atmospheric dimensional equations are formulated on an f plane using boundary layer temperature is assumed to be in equi- classical scaling and are then linearized about a background librium with the underlying SST, and the stress results Ekman spiral due to a prescribed, geostrophic wind. from a one-dimensional momentum budget in the The active layer of depth h is capped by an inversion boundary layer in response to the frontally induced with potential temperature jump DQ and a reduced gravity 0 g 5 (DQ/Q0)g,whereg is Earth’s gravitational accelera- Q 1 This effect is called ‘‘buoyancy shutdown’’ in the oceanic bot- tion, and 0 is a reference boundary layer potential tem- tom boundary layer (MacCready and Rhines 1991) and drastically perature. This active layer is forced by prescribed, constant, reduces bottom friction (Benthuysen 2010). barotropic, geostrophic wind with a lateral scale far larger 3358 JOURNAL OF THE ATMOSPHERIC SCIENCES VOLUME 72 than the frontal circulation and sea surface temperature A E 5 , (3) T. We consider the system in steady state, restricting fH2 time scales to longer than the maximum of an inertial period, the spindown time scale (see below), and the with A being the vertical exchange coefficient that, in thermal adjustment time of the layer. general, is a function of s, and by the reduced gravity Equations and variables are nondimensionalized us- pffiffiffiffiffiffiffiffi + ing the Rossby radius g0H/f as a horizontal scale, with g 5 1 2Q (4) f the Coriolis frequency, the mean inversion height H as pffiffiffiffiffiffiffiffi the vertical scale, and the gravity wave speed g0H that includes the impacts of the varying temperature in as the horizontal velocity scale (Table A1).
Details
-
File Typepdf
-
Upload Time-
-
Content LanguagesEnglish
-
Upload UserAnonymous/Not logged-in
-
File Pages22 Page
-
File Size-