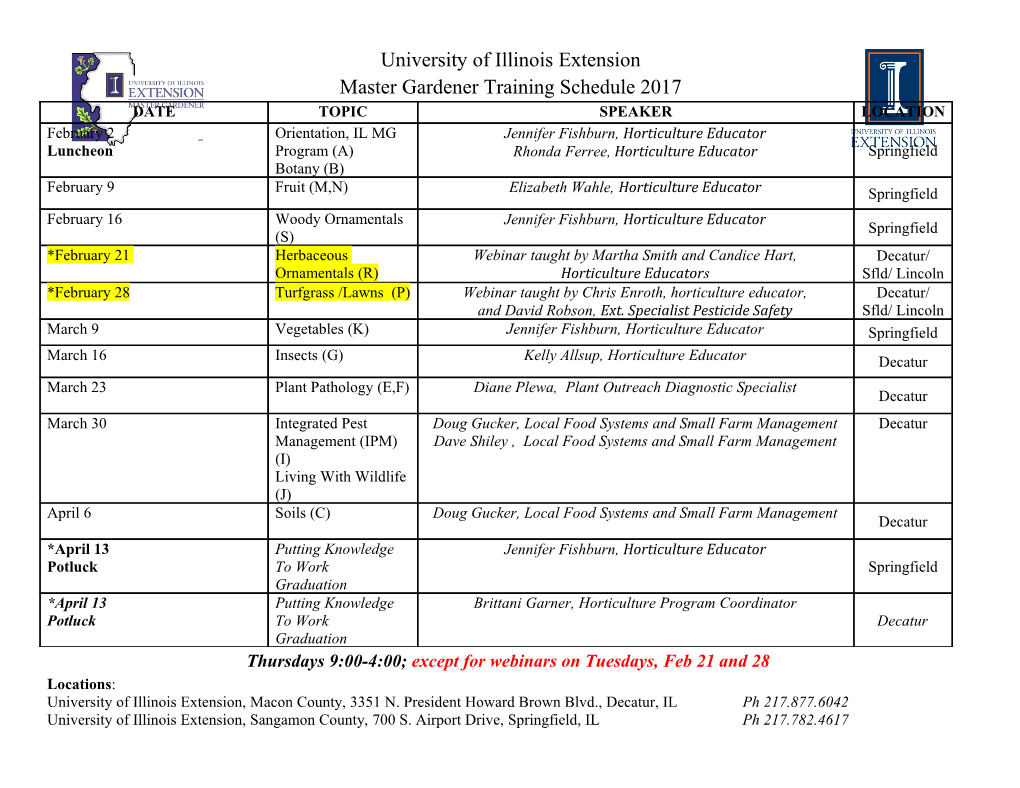
Synthesis and Reactivity of Medium-Bridged Twisted Lactams by Michal L. Szostak M.Sc., Wroclaw Medical University, 2005 Submitted to the Department of Medicinal Chemistry and the Faculty of the Graduate School of the University of Kansas in partial fulfillment of the requirements for the degree of Doctor of Philosophy Thesis Committee _______________________________ (Chairperson) _______________________________ _______________________________ _______________________________ _______________________________ Date defended: ____________________ The Dissertation Committee for Michal L. Szostak certifies that this is the approved version of the following dissertation: Synthesis and Reactivity of Medium-Bridged Twisted Lactams Thesis Committee _______________________________ (Chairperson) _______________________________ _______________________________ _______________________________ _______________________________ Date defended: ____________________ ii Abstract The research presented herein describes the development of synthetic methods to one-carbon bridged twisted amides and the study of properties of one-carbon bridged lactams. Initial investigations focused on electrostatic cation–π and cation–n interactions as regiochemistry controlling feature of the intramolecular Schmidt reaction to provide access to one-carbon bridged amides. In cases where the reactive conformation of the azidohydrin intermediate is locked, the selectivity of the reaction depends on the electron density of an aromatic ring oriented in 1,3-diaxial relationship with regard to the diazonium cation. However, a placement of a heteroatom in the α-position to the ketone permits the synthesis of otherwise unsubstituted bridged amides from conformationally flexible ring systems. Also, described is the development of a general method of synthesis of one-carbon bridged amides relying on a transannular cyclization strategy. Next, experiments directed towards investigation of unusual properties of distorted amides are presented. One-carbon bridged lactams display superior to other bridged amides levels of hydrolytic stability. These lactams participate in a number of interesting and potentially useful reactions unknown to traditional amide bonds, including synthesis of remarkably stable tetrahedral intermediates and a direct conversion into bridged spiro-epoxyamines. The influence of the amide bond geometry on reactivity of distorted lactams is also discussed. iii For Magda iv Acknowledgements First and foremost, I would like to thank my advisor, Professor Jeffrey Aubé for his advice and support over the past few years. I will always be grateful for the opportunity to work in his laboratory. The satisfaction I experienced as a graduate student is directly related to his scholarly excellence in research, scientific writing and presentation. There is no doubt that any success I may find in my future career will arise from mentorship I received in Jeff’s lab. I would like to thank Professors Apurba Dutta, Sunil David, Paul Hanson, Michael Rubin and Helena Malinakova for invaluable instruction in the classroom and for their time and input as members of my thesis committee. I would like to thank all my colleagues in the Aubé group with whom I have had an opportunity to work with. It has been a wonderful experience to be a part of the Aubé group over these years. I would like to thank my undergraduate advisor, Professor Stanislaw Ryng for instilling in me passion for organic medicinal chemistry, and for his interest in my research. I am deeply grateful to my family for all their endless support, love and understanding; they have made this thesis possible, and for that I thank them most wholeheartedly. Finally, I would like to give my deepest thanks to my wife. Her love and support for me have been limitless. This thesis is dedicated in her honor. v Table of Contents Page Abstract iii Acknowledgements v List of Figures viii List of Tables x Chapter 1. Introduction 1 Amide bond 1 Distorted amide bonds 2 Types of distorted amides 5 Synthesis of bridged amides 9 Schmidt reaction and bridged lactams 23 Schmidt reaction and electrostatic interactions 28 Reactivity of bridged amides 32 Transannular amine-carbonyl interactions 39 Tetrahedral intermediates 41 Chapter 2. Synthesis of Medium-Bridged Amides 43 Pyramidalization at nitrogen in Schmidt reaction 44 Cation−π control of regiochemistry in Schmidt reaction 50 Cation−n control of regiochemistry in Schmidt reaction 61 vi Transannular cyclization strategy 77 Bicycle opening 84 Fukuyama amine synthesis 87 RCM cyclizations 98 Oxidative cyclization approach 115 Summary 118 Chapter 3. Reactivity of Medium-Bridged Twisted Amides 120 Hydrolytic stability of medium-bridged twisted amides 121 Proximity effects in nucleophilic addition reactions 135 Corey-Chaykovsky reaction of bridged amides 148 Synthesis and rearrangement of a bridged thioamide 162 Bridged exocyclic enamine 170 Miscellaneous reactions (comparison of distortion parameters) 174 Summary 180 Chapter 4. Experimental Section 181 References 416 vii List of Figures Page Figure 1. Electron delocalization in amides. 1 Figure 2. Twisting of the amide bond by rotation around the N–C(O) 2 bond. Figure 3. a) Steric repulsion and b) conformational restriction of amide 4 bonds. Figure 4. Examples of distorted amides resulting from steric repulsion. 8 a) N-acylpyrroles, b) N-acylthiazolidine-2-thiones, c) 2,5- dithioglycoluril, d) N-benzyloxycarbonylamide. Figure 5. a) Difficulties in cyclization to a bridged amide. b) Ease of 11 synthesis of its geometrical isomer. c) Resonance structures in strained penicillin antibiotics. Figure 6. Bridged bicyclic sultams. 17 Figure 7. Reactive intermediates in the first and the second generation 28 synthesis of stenine. Figure 8. a) Examples of N…C=O interactions. b) Compounds whose 40 X-ray structures provided the basis for Bürgi-Dunitz trajectory (the trajectory is shown in the box). Figure 9. a) Reaction of carboxylic acid derivatives with nucleophiles. 42 b) Examples of isolable tetrahedral adducts. viii Figure 10. Unproductive azidohydrin intermediates arising from 46 cyclohexanone bearing equatorial azidoalkyl tether (bonds + antiperiplanar to N2 marked in bold). Figure 11. X-ray structure of bridged amide 38. 60 Figure 12. Types of bridged amides. 77 Figure 13. Ring strain energy (kcal/mol) in bridgehead olefins analogous 83 to 1-aza-2-adamantanone, 2-qunuclidone, [4.3.1], [5.3.1] and [3.3.1] one-carbon bridged amides. Traditional olefin and saturated analogue are also shown for comparison. Figure 14. Fukuyama amine synthesis in the synthesis of medium-sized 87 heterocycles. Figure 15. Amides used in the study of hydrolytic stability of one- 122 carbon bridged lactams. 13 Figure 16. C NMR spectra of compound 229 in (a) CDCl3, (b) 1:1 126 D2O:THF-d8, (c) 1:6 DCl (1 N in D2O)/THF-d8 and (d) 1:6 NaOD (1 N in D2O)/THF-d8. 13 Figure 17. C NMR spectra of lactam 58 in (a) CDCl3 and (b) D2O. 133 Figure 18. (a) Examples of isolable aminoepoxides. (b) Common 148 decomposition pathway of unmodified aminoepoxides. Figure 19. Biologically relevant thioamides. 162 Figure 20. Examples of bridged enamines and their derivatives. 170 ix List of Tables Page Table 1. Structural parameters and hydrolysis rate constants of 2- 14 quinuclidone derivatives. Table 2. Effect of Lewis Acid on Product Distribution with Azide 1. 47 Table 3. Effect of Temperature on Product Distribution with Azide 1. 48 Table 4. Effect of Solvent on Product Distribution with Azide 1. 48 Table 5. Preparation of Azides 20-26. 51 Table 6. Optimization of Product Distribution in Schmidt Reaction 52 with Azide 20. Table 7. Cation–π Directed Synthesis of Fused and Bridged Lactams. 53 Table 8. Influence of Lewis Acid Stoichiometry on Product 55 Distribution in Cation–π Directed Schmidt Reaction. Table 9. Schmidt Reactions of α-Carbonyl Substituted Azides. 58 Table 10. Synthesis of Bridged and Fused Lactams Utilizing Cation–n 65 Effects. Table 11. Effect of Substituents and Ring Sizes on Cation–n Directed 69 Schmidt Reaction. Table 12. Acid Influence on Bridged/Fused Lactam Ratios in Cation–n 71 Directed Schmidt Reactions. Table 13. Transannular Closure to Bridged Amides. 82 x Table 14. ESI MS Experiments with lactam 153. 93 Table 15. Optimization of RCM for the Formation of 9-Membered 102 Ring. Table 16. Synthesis of Bridged Lactams via RCM/Transannular 107 Cyclization Sequence. Table 17. Transannular Cyclization to a [5.3.1] Ring System. 109 Table 18. Transannular Cyclization to a [4.4.1] Ring System. 110 Table 19. Comparison of Spectroscopic Properties of Saturated 114 Lactams. Table 20. Extraction Studies of Lactam 229. 124 Table 21. 13C NMR Carbonyl Shifts of Lactam 229 and Its Derivatives. 127 Table 22. ESI MS Experiments with Lactam 229. 129 Table 23. Extraction Studies of Bicyclic Lactams. 132 Table 24. Hydride Addition to One-Carbon Bridged Amides. 136 Table 25. Reduction of Lactam 34. 138 Table 26. Organometallic Addition to Bicyclic Amides. 141 Table 27. Organometallic Addition to Tricyclic Amides. 143 Table 28. Corey-Chaykovsky Reaction with Amide 34. 150 Table 29. Scope of the Corey-Chaykovsky Reaction. 152 Table 30. Reactions of 275 under Lewis Acidic Conditions. 158 Table 31. Additional Reactions of Amino-epoxide 275. 160 Table 32. Influence of Additional Reaction Conditions on Thionation of 166 xi 34. Table 33. Spectroscopic Properties of Bridged and Planar Lactams and 168 Thiolactams in Comparison with Structurally-Related Ketones and Thioketones. Table 34. Optimization of
Details
-
File Typepdf
-
Upload Time-
-
Content LanguagesEnglish
-
Upload UserAnonymous/Not logged-in
-
File Pages457 Page
-
File Size-