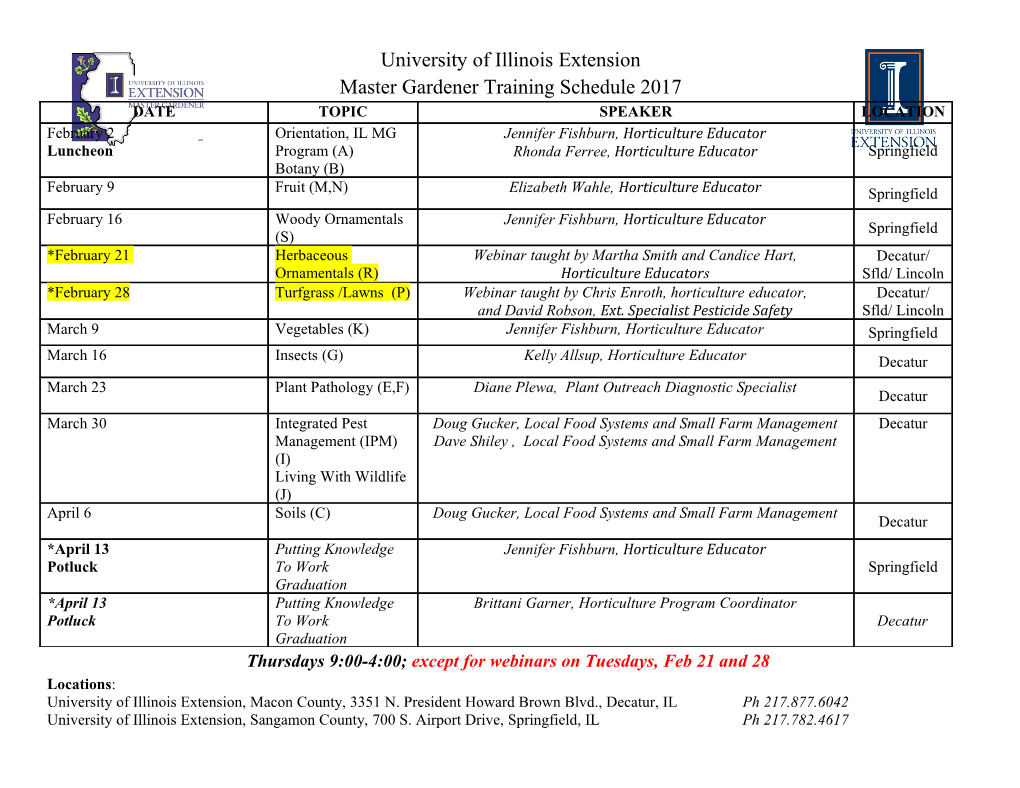
COMPUTATIONAL MODELING OF PLUME DYNAMICS IN MULTIPLE PULSE LASER ABLATION OF CARBON A Dissertation Presented to The Graduate Faculty of The University of Akron In Partial Fulfillment of the Requirements for the Degree Doctor of Philosophy K Kedar Ashok Pathak August, 2008 COMPUTATIONAL MODELING OF PLUME DYNAMICS IN MULTIPLE PULSE LASER ABLATION OF CARBON Kedar Ashok Pathak Dissertation Approved: Accepted: Advisor Department Chair Dr. Alex Povitsky Dr. Celal Batur Committee Member Dean of the College Dr. Erol Sancaktar Dr. George K. Haritos Committee Member Dean of the Graduate School Dr. Gerald Young Dr. George R. Newkome Committee Member Date Dr. Minel Braun Committee Member Dr. Scott Sawyer ii ABSTRACT The flow field induced by the ablation plume in the presence of background gas is simulated numerically. The study of plume flow that occurs in laser ablation is impor- tant for it can yield information on ablation process itself and the properties of end product for which the ablation is carried out. Unsteady compressible axisymmetric Navier-Stokes equations govern the plume flow. The major challenge involved, even in this simplified model of plume dynamics, is twofold: (i) the time scale of simu- lation spans six orders of magnitude, from nanosecond to millisecond, and (ii) the high nonlinearity of governing equations because of high pressure, temperature and injection velocity of plume. A computational model is developed that can account for the entire range of time scale and high nonlinearity. This model is a combination of numerical methods and includes multi-time step and multi-size grid technique. The uniqueness of model lies in choosing the combination of numerical methods and han- dling multi-size grid interface in a conservative way. The combination of numerical methods is decided after comparing the results of few numerical methods for a single plume. The plume dynamics for single plume is explained with the help of proposed post-processing model based on vorticity dynamics. The model not only helps in understanding the expansion dynamics of plume but also provides quantitative com- parison amongst numerical methods. The validity of nano-to-micro second range iii viscous and inviscid models of plume dynamics is discussed by means of evaluation of source terms in the vorticity transport equation. The role of turbulence is evaluated by millisecond-scale modeling of plume expanding in surrounding furnace gas with imposed turbulent gust. The results for multiple plumes typical for real life ablation are presented and discussed. Shielding of laser beam by previously ejected plume in multiple laser hits is important because it changes energy deposition of incident laser pulse at the target surface and in turn influences the ablation dynamics and amount of material removed. To account for this shielding effect, shielding models are developed and implemented. The quantity of ablated mass due to the shielding effect is evaluated. Ionization of carbon plume and its impact on plume dynamics and shielding is studied. An iterative procedure is developed to determine the local equilibrium temperature affected by ionization. It is shown that though shielding due to the presence of ionized particles in carbon plume is small, the effect of ionization on plume dynamics can be considerable. Shielding effect is calculated for laser pulses with different time interval between pulses. The effect of high temperature and low density of plume are controversary and cause shielding behavior to be non-monotonic with pulse number. It is shown that the non-monotonic dependence of the delivered laser energy with the pulse number and the difference in shielding characteristics between planar and axisymmetric formulations increase with the time duration between two consecutive pulses. The developed numerical methodology is employed to study the heat transfer modulation between the Thermal Protection Shield (TPS) and the gas flow occurring because of ejection of under-expanded pyrolysis gases through the cracks in the TPS in hypersonic flight. The simulations are performed for an axisymmetric bluff body flying at Mach 7. The influence of the geometry of the TPS on heat transfer pattern iv is studied for two representative shapes. The results are presented for three different flight altitudes (low-ground level, moderate-20km and high-30km). At the low alti- tude the plume pressure is lower than the pressure behind the detached front shock wave and the plume propagates slowly along the wall surface. At high and moder- ate altitudes, the plume path and consequently, convective heat transfer between the TPS and the plume depends on the plume interaction with the bow shock wave. The effect of viscosity for the plume injection conditions and free stream Mach number considered is found to be negligible at simulated altitudes. However, the effect of initial pressure of pyrolysis gas on the plume dynamics is significant. The presence of the blast wave associated with under-expanded plume alters the heat transfer and increases mixing. Finally, the enhanced heat transfer caused by the emergence of multiple pyrolysis plumes is investigated. v ACKNOWLEDGEMENTS I would like to thank Dr. Alex Povitsky for giving me the opportunity to work with him. His excellent support and expert guidance has made the seemingly wide topic of modeling laser ablation practical for a novice like me. I am also thankful to Dr. Erol Sancaktar, Dr. Gerald Young, Dr. Scott Sawyer and Dr. Minel Braun for their review of the presented material and sugges- tions. I highly value their critical comments and suggestions for this work. The work content of Chapter IV was partially supported by the Air Force Office of Scientific Research (AFOSR) through research grant FA9550-07-1-0457. Dr. Datta Gaitonde's comments for this chapter helped to improve the material of this chapter which is gratefully acknowledged. Finally, it remains to thank all my colleagues for their valuable direct and indirect help from point to point through out the years of this research. vi TABLE OF CONTENTS Page LIST OF TABLES . x LIST OF FIGURES . xi CHAPTER I. INTRODUCTION . 1 1.1 Research Motivation . 2 1.2 Previous Research . 5 1.3 Multiple Plume Ejections . 21 1.4 Objectives . 22 II. MATHEMATICAL MODELING . 26 2.1 Set-up of Problem . 26 2.2 Governing Equations . 28 vii 2.3 Treatment of Boundary Conditions . 30 2.4 Modeling of Turbulence . 31 2.5 Numerical Methodology and Validation . 33 2.6 Ionization and Shielding . 44 2.7 Multi-Time Step Modeling . 47 III. RESULTS AND DISCUSSION FOR LASER ABLATION MOD- ELING . 52 3.1 Single gamma versus Two gamma . 53 3.2 Inviscid, Viscous and Turbulence Plume Modeling . 54 3.3 Dynamics of Vorticity . 62 3.4 Multiple Plumes . 67 3.5 Shielding Effect . 71 3.6 Multi-time Step Modeling . 73 3.7 Axisymmetric vs. Planar Plumes . 77 3.8 Ionization Effect . 86 IV. APPLICATION OF DEVELOPED MODEL IN MODELING PLUMES GENERATED BY LOCAL INJECTION OF CAR- BON ABLATION PRODUCTS IN HIGH-SPEED FLIGHT . 91 viii 4.1 Background and Previous Research . 91 4.2 Description of Model . 94 4.3 The Effect of the Flight Altitude on Plume Dynamics and Heat Transfer . 100 4.4 Heat Transfer for the Range of Plume Injection Pressures . 108 4.5 Heat Transfer for Multiple Plume Injections . 110 4.6 Heat Transfer for Different TPS Shapes of Comparable Sizes . 112 V. SUMMARY AND FUTURE SCOPE . 115 5.1 Future Scope . 121 BIBLIOGRAPHY . 123 APPENDICES . 133 APPENDIX A. MULTI-SIZE MESH FOR MULTI-TIME STEP MODELING . 134 APPENDIX B. GRID CONVERGENCE . 137 APPENDIX C. IONIZATION PREDICTION . 138 APPENDIX D. TWO-TEMPERATURE MODELING . 140 ix ix LIST OF TABLES Table Page 1.1 A survey of numerical methods for LIP simulation in laser ablation . 21 3.1 Summary of operating parameters of the process being simulated . 52 4.1 Flight regimes and parameters of surrounding gas . 95 4.2 Plume injection parameters . 95 4.3 Value of normalization parameter at different altitude . 97 B.1 L1-Norm of the errors for Numerical solution of ut + uux = 0; u(x; 0) = sin(πx) ............................ 137 x LIST OF FIGURES Figure Page 1.1 Schematic of experimental set-up for laser ablation . 6 1.2 In situ imaging and spectroscopic diagnostic investigations of SWNT's growth (Taken from Ref. [1] with author's permission) . 8 2.1 Problem schematic a) process of laser ablation showing the expand- ing plume and propagating shock wave and b) set-up of geometry and boundary conditions; b1 is inflow, b2-b3 is solid wall, b4 is outflow and b5 is target condition . 27 2.2 Fluctuating velocity components a) Horizontal b) Vertical . 33 2.3 Flow chart of the numerical code . 34 2.4 Structure of the solution of the considered Riemann problem . 38 2.5 Density contours for the double Mach reflection problem, ∆x = ∆y = 1=120: a) the Godunov method b) the Relaxing TVD method and c) the ENO-Roe method . 39 2.6 Density contours for the forward facing step problem, ∆x = ∆y = 1=80: a) the Godunov method b) the Relaxing TVD method and c) the ENO-Roe method . 40 xi 2.7 Initial/boundary conditions for shock flowing over backward facing step problem . 41 2.8 Density contours for shock flowing over backward facing step prob- lem, ∆x = ∆y = 1=300: a) the Godunov method and b) the Relax- ing TVD method c) the ENO-Roe method . 41 2.9 Normalized velocity distributions as obtained for boundary-layer type flow by a) the Godunov method and b) the ENO-Roe Scheme . 43 2.10 Multi-size mesh for multi-time step modeling: a) schematic showing grid sizes for coarse and fine girds (L and D are furnace length and diameter, respectively) and b) computational domain with bound- ary conditions .
Details
-
File Typepdf
-
Upload Time-
-
Content LanguagesEnglish
-
Upload UserAnonymous/Not logged-in
-
File Pages158 Page
-
File Size-