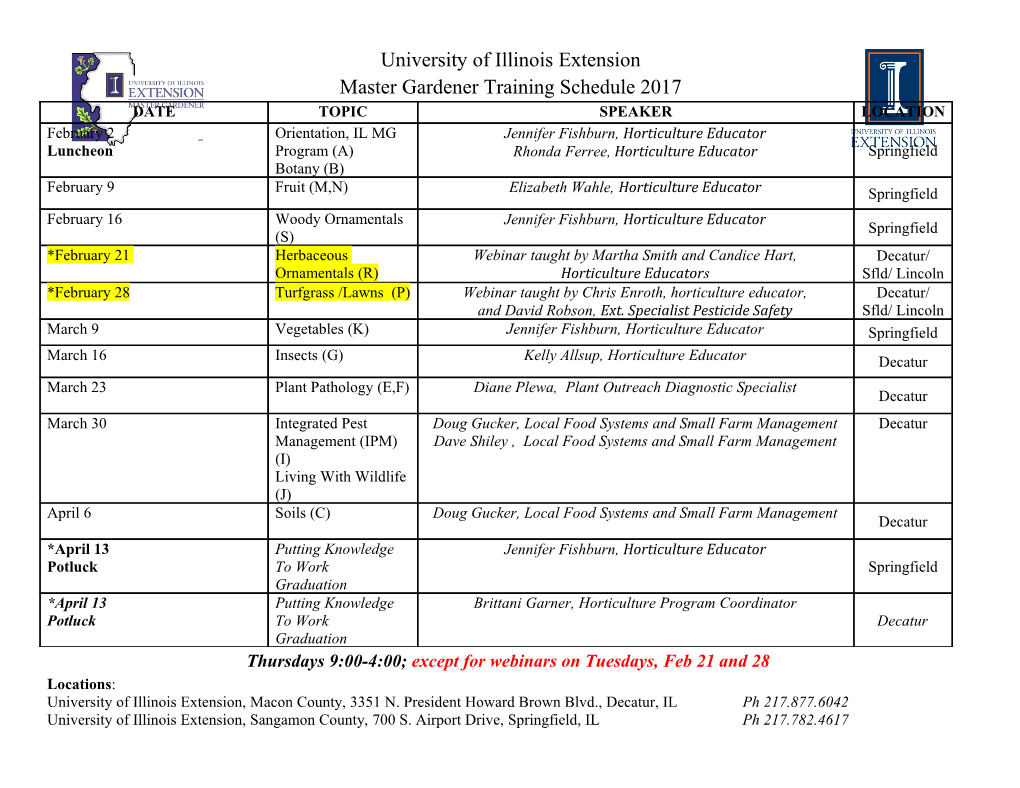
Chapter 6 The Fossil Record of Shell-Breaking Predation on Marine Bivalves and Gastropods RICHARD R. ALEXANDER and GREGORY P. DIETL I. Introduction 141 2. Durophages of Bivalves and Gastropods 142 3. Trends in Antipredatory Morphology in Space and Time .. 145 4. Predatory and Non-Predatory Sublethal Shell Breakage 155 5. Calculation ofRepair Frequencies and Prey Effectiveness 160 6. Prey Species-, Size-, and Site-Selectivity by Durophages 164 7. Repair Frequencies by Time, Latitude, and Habitat.. 166 8. Concluding Remarks 170 References 170 1. Introduction Any treatment of durophagous (shell-breaking) predation on bivalves and gastropods through geologic time must address the molluscivore's signature preserved in the victim's skeleton. Pre-ingestive breakage or crushing is only one of four methods of molluscivory (Vermeij, 1987; Harper and Skelton, 1993), the others being whole­ organism ingestion, insertion and extraction, and boring. Other authors in this volume treat the last behavior, whereas whole-organism ingestion, and insertion and extraction, however common, are unlikely to leave preservable evidence. Bivalve and gastropod ecologists and paleoecologists reconstruct predator-prey relationships based primarily on two, although not equally useful, categories of pre-ingestive breakage, namely lethal and sublethal (repaired) damage. Peeling crabs may leave incriminating serrated, helical RICHARD R. ALEXANDER • Department of Geological and Marine Sciences, Rider University, Lawrenceville, New Jersey, 08648-3099. GREGORY P. DIETL. Department of Zoology, North Carolina State University, Raleigh, North Carolina, 27695-7617. Predator-Prey Interactions in the Fossil Record, edited by Patricia H. Kelley, Michal Kowalewski, and Thor A. Hansen. Kluwer Academic/Plenum Publishers, New York, 2003. 141 142 Chapter 6 fractures in whorls of high-spired gastropods (Bishop, 1975), but unfortunately most lethal fractures are far less diagnostic of the causal agent and often indistinguishable from abiotically induced, taphonomic agents ofshell degradation. Published research has focused on gastropod and bivalve shell repairs in the fossil record (Vermeij, 1987), although reliance on sublethal damage to reconstruct predator­ prey interactions is not without complications. Sublethal, repaired shell damage is commonly inversely related to lethal, unrepaired shell damage (Vermeij, 1982a), a relationship that may lead to ambiguous reconstruction of predation intensity (Kowalewski et al., 1997; Leighton, in press). As strength of the predator's crushing elements increase, or, concurrently, strength of the prey shell decreases, lethal damage increases, whereas sublethal damage decreases. Furthermore, a clam victim may sustain sublethal tissue damage, such as siphon nipping (Peterson and Quammen, 1982) by fish or crabs that may not break the shell. No evidence, other than slowed growth rate (Coen and Heck, 1991) records the predatory attempt. Furthermore, time-averaging (Cadee et al., 1997) and high within-habitat variability (Schmidt, 1989) may complicate inferences on prey evolution derived from the record of shell repairs, particularly if sample sizes are small. Although predation intensity cannot be inferred directly from bivalve (Dietl et al., 2000; Alexander and Dietl, 2001) or gastropod (Vermeij et al., 1981; Schindel et at., 1982) frequencies of breakage-induced shell repair, injury to the shell is a necessary condition for selection for defensive adaptations (Vermeij, 1982b). Utility of repair frequencies to tests of the escalation hypothesis in marine molluscs is central to this review; escalation involves adaptation to enemies, stressing the importance of predation as a driving force in evolution. Vermeij et at. (1981, p. 1024) postulated that: selection favoring the evolution of breakage-resistant shells can occur only if individuals in a population reproduce after they have suffered nonlethal shell-breaking attacks. If all breakage were lethal, there would be no selection between weak and strong shell variants and no shells would show the scars that record nonlethal injury. High frequencies of sublethal damage imply that the shell, together with other defenses, is effective in protecting the gastropod [or bivalve] against locally prevailing, shell­ breaking agents. The higher the frequency of sublethal shell damage, the greater is the likelihood that selection will maintain or enhance shell armor. 2. DUfophages of Bivalves and Gastropods Vermeij (1987) traced the evolutionary history of predators capable of shell crushing as one line of evidence to support his hypothesis that breakage became a more important component of natural selection for shallow-water, marine, shelled invertebrates through the Phanerozoic. Vermeij (1987) reasoned that if individuals are exposed to increasingly greater risks from their enemies over geologic time, a temporal trend towards greater reliance on methods of rapid prey subjugation would be expected. The developing emphasis on breakage, a rapid predation method, through the Phanerozoic, agrees with the prediction that high-energy modes ofpredation are favored as risks to the predators themselves increase (Vermeij, 1987). Shell Breaking of Bivalves and Gastropods 143 The dominant predators responsible for shell damage in marine gastropods and bivalves over geologic time include a varied array of clawed and jawed higher taxa. Marine Phanerozoic life has been apportioned among three "evolutionary faunas," namely the Cambrian, Paleozoic, and Modem (Sepkoski, 1984). Each "fauna" had its shell breakers. Potential shell breakers that dominated the Cambrian fauna were the anomalocarids and Sidneyia (Conway Morris and Whittington, 1979; Nedin, 1999). Sidneyia is preserved with brachiopod fragments in its stomach contents (Conway Morris and Whittington, 1979) and could have been molluscivorous. The Mid­ Paleozoic revolution in durophagy (Signor and Brett, 1984) represented the first large­ scale diversification of shell-breaking vertebrate and invertebrate predators. Phyllocarids (Rolfe, 1969), belotelsonid (Schram, 1981), and pygocephalomorph eumalacostracans are among invertebrate shell crushers. Signor and Brett (1984) excluded Silurian eurypterids such as Pterygotus from their tally of durophages because they lacked calcified chelicerae. Nevertheless, Vermeij (1987) conceded that pterygotid eurypterids, with their well-worn, pointed teeth on their massive chelicerae, could have been durophagous. Signor and Brett (1984) also excluded nautiloids as Mid-Paleozoic shell breakers because they possessed a non-calcified jaw, or rhyncholite, that may have been capable of crushing calcareous exoskeletons. However, several investigators attributed scars in strophomenid (Alexander, 1986) or productid (Sarycheva, 1949; Elliott and Brew, 1988) brachiopods to nautiloids, despite the probability that the beaks were chitinous. Horny (1997) likewise attributed repair in the Ordovician mollusc Sinuitopsis neglecta to nautiloids. Ammonoids are also possible durophages on Paleozoic gastropods and bivalves, although Signor and Brett (1984) were uncertain of their diet and excluded them as durophages. Bond and Saunders (1989) attributed sublethal damage in Late Mississippian ammonoids to attacks by other ammonoids. Placoderm and ptyctodont fishes and chondrichthyans comprise the bulk of Mid­ Paleozoic durophagous taxa (Signor and Brett, 1984; Brett and Walker, in press), although some Late Mississippian sharks are disputed shell crushers. Cladodontids have been implicated as shell piercers (Brunton, 1966; Mapes and Hansen, 1984; Mapes et ai, 1989), but Signor and Brett (1984) doubted that these sharks were shell crushers. Equal spacing and geometry of punctures occasionally preserved in Carboniferous brachiopod valves suggest that cladodontids could have broken shelled prey. Chimaeras also have been implicated as shell crushers of Permian bivalves (Brett and Walker, in press). In addition, chondl"ostean fishes (Doryopteridae) may have been durophagous (Brett and Walker, in press). The modem (Mesozoic to Recent) molluscan fauna was preyed upon by an even larger diversity of durophages than the Paleozoic fauna (Vermeij, 1977); this interval represents the second major episode of diversification of shell-breaking predators. Shell crushers arguably capable of sublethal, repairable damage on marine bivalves and gastropods, during the Mesozoic, were reviewed by Vermeij (1977, 1987). Triassic shell crushers included placodont reptiles (von Huene, 1956; Walker and Brett, in press). Pycnodontid reef fishes evolved shell-crushing teeth in the Jurassic (Walker and Brett, in press). Dominant shell crushers of the Jurassic and Cretaceous were ptychodid sharks and batoid rays and skates (Walker and Brett, in press). Kauffman (1972) implicated Ptychodus predation on Cretaceous Inoceramus bivalves. Other vertebrate shell crushers during the Cretaceous include chelonoid turtles (Hirayama, 1997) and 144 Chapter 6 globodentine mosasaurs (Massare, 1987). Among invertebrates, the homarid and palinurid lobsters (Harper, 1991; George and Main, 1968) evolved in the Triassic, and brachyuran (Xanthidae; Late Cretaceous) and pagurid crustaceans first appeared in the Jurassic. It is debatable whether these early forms were capable of crushing shells (Vermeij,1993). Invertebrate taxa that evolved a durophagous habit in the Cenozoic include the brachyuran crabs (with functionally differentiated claws: e.g., Portunidae and Cancridae) and neogastropods (Fasciolariidae,
Details
-
File Typepdf
-
Upload Time-
-
Content LanguagesEnglish
-
Upload UserAnonymous/Not logged-in
-
File Pages36 Page
-
File Size-