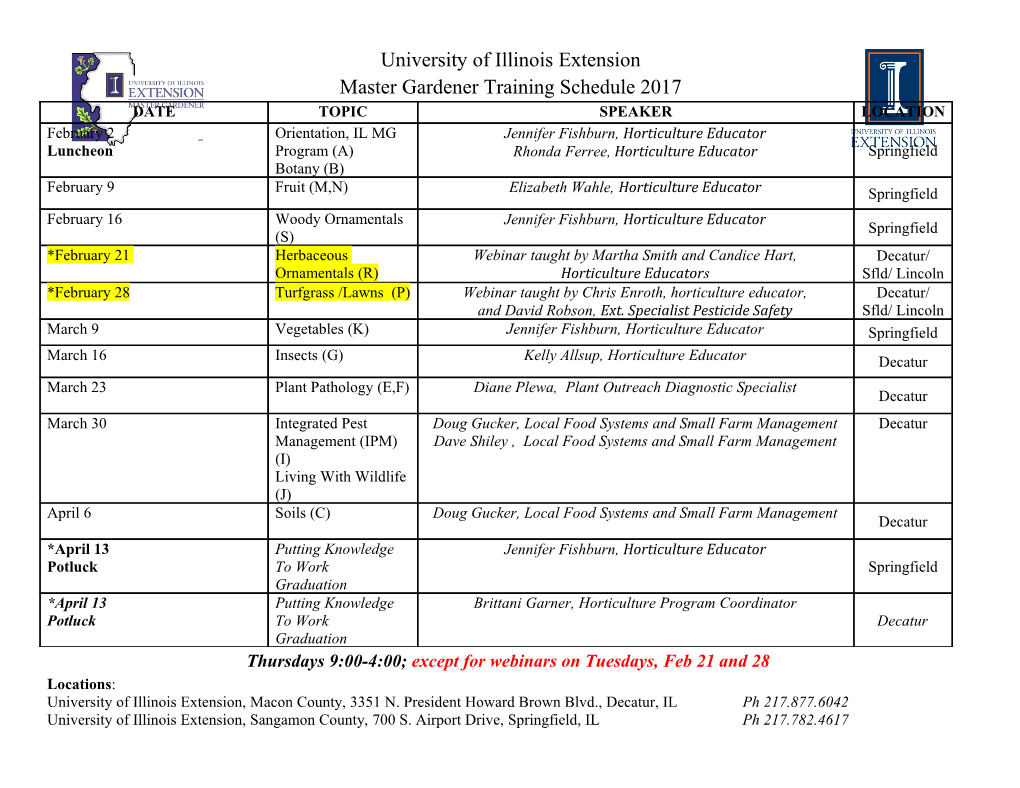
PUBLICATIONS Global Biogeochemical Cycles RESEARCH ARTICLE Contribution of regional sources to atmospheric methane 10.1002/2015GB005300 over the Amazon Basin in 2010 and 2011 Special Section: Chris Wilson1,2,3, Manuel Gloor3, Luciana V. Gatti4, John B. Miller5,6, Sarah A. Monks2,7,8, Trends and Determinants of Joey McNorton1,2, A. Anthony Bloom9, Luana S. Basso4, and Martyn P. Chipperfield1,2 the Amazon Rainforests in a Changing World, A Carbon 1National Centre for Earth Observation, University of Leeds, Leeds, UK, 2School of Earth and Environment, University of Cycle Perspective Leeds, Leeds, UK, 3School of Geography, University of Leeds, Leeds, UK, 4Instituto de Pesquisas Energéticas e Nucleares, Comissao Nacional de Energia Nuclear, Atmospheric Chemistry Laboratory, Sao Paulo, Brazil, 5Global Monitoring Key Points: Division, Earth System Research Laboratory, National Oceanic and Atmospheric Administration, Boulder, Colorado, USA, • Amazon Basin emits 31-42 Tg 6Cooperative Institute for Research in Environmental Sciences, University of Colorado Boulder, Boulder, Colorado, USA, (methane)/yr in 2010-2011 7 • Basin contributes significantly (up to Now at Chemical Sciences Division, Earth System Research Laboratory, National Oceanic and Atmospheric Administration, 8 7.5%) to global methane emissions Boulder, Colorado, USA, Now at Cooperative Institute for Research in Environmental Sciences, University of Colorado • Total noncombustion basin emissions Boulder, Boulder, Colorado, USA, 9Jet Propulsion Laboratory, California Institute of Technology, Pasadena, California, USA were similar in 2010 and 2011 We present an assessment of methane (CH ) atmospheric concentrations over the Amazon Supporting Information: Abstract 4 • Figure S1 and Table S1 Basin for 2010 and 2011 using a 3-D atmospheric chemical transport model, two wetland emission models, and new observations made during biweekly flights made over four locations within the basin. We attempt to Correspondence to: constrain basin-wide CH4 emissions using the observations, and since 2010 was an unusually dry year, we C. Wilson, assess the effect of this drought on Amazonian methane emissions. We find that South American emissions [email protected] contribute up to 150 ppb to concentrations at the sites, mainly originating from within the basin. Our atmospheric model simulations agree reasonably well with measurements at three of the locations Citation: (0.28 ≤ r 2 ≤ 0.63, mean bias ≤ 9.5 ppb). Attempts to improve the simulated background CH concentration Wilson, C., M. Gloor, L. V. Gatti, J. B. Miller, 4 S. A. Monks, J. McNorton, A. A. Bloom, through analysis of simulated and observed sulphur hexafluoride concentrations do not improve the model L. S. Basso, and M. P. Chipperfield (2016), performance, however. Through minimisation of seasonal biases between the simulated and observed Contribution of regional sources to atmospheric concentrations, we scale our prior emission inventories to derive total basin-wide methane atmospheric methane over the Amazon Basin in 2010 and 2011, Global emissions of 36.5–41.1 Tg(CH4)/yr in 2010 and 31.6–38.8 Tg(CH4)/yr in 2011. These totals suggest that the Biogeochem. Cycles, 30, doi:10.1002/ Amazon contributes significantly (up to 7%) to global CH4 emissions. Our analysis indicates that factors other 2015GB005300. than precipitation, such as temperature variations or tree mortality, may have affected microbial emission rates. However, given the uncertainty of our emission estimates, we cannot say definitively whether Received 5 OCT 2015 Accepted 3 FEB 2016 the noncombustion emissions from the region were different in 2010 and 2011, despite contrasting Accepted article online 6 FEB 2016 meteorological conditions between the two years. 1. Introduction Methane (CH4) is a powerful greenhouse gas that is emitted into the atmosphere from a variety of anthropo- genic and natural sources. As the second most important long-lived atmospheric greenhouse gas [Myhre et al., 2013], it has an influential role on the Earth’s climate and on the oxidizing capacity of the troposphere [Prather et al., 2001]. Natural wetlands, the largest single source of methane into the atmosphere (110–284 Tg (CH4)/yr) [Matthews and Fung, 1987; Kirschke et al., 2013], make up around 20–40% of the total natural and anthropogenic sources of atmospheric methane. Due to the sensitivity of wetland emissions to climate fac- tors such as temperature and precipitation, these emissions may significantly affect the interannual variation of atmospheric CH4 [Fung et al., 1991; Bousquet et al., 2006; Kirschke et al., 2013; Pison et al., 2013]. Similarly, CH4 emissions from biomass burning exhibit large interannual variability due to the dependence of fire occurrence and intensity on meteorological conditions [van der Werf et al., 2010]. Due partly to the variable contribution of these natural sources, along with variations in climate [Rigby et al., 2008] and the relatively short lifetime of CH4 (9–11 years) [Prinn et al., 2005; Fiore et al., 2008; Prather et al., 2012], the annual growth ©2016. The Authors. fi This is an open access article under the rate of atmospheric methane varies signi cantly over short time scales (e.g., a few years). After a period of terms of the Creative Commons growth throughout the 1990s, the atmospheric growth rate of CH4 averaged approximately zero for the Attribution License, which permits use, period 2000–2006, after which it once again rose steadily until the present [Dlugokencky et al., 2011; Nisbet distribution and reproduction in any medium, provided the original work is et al., 2014]. Total methane emissions, as well as the partitioning of emissions between different source types, properly cited. are not well constrained, and although several hypotheses have been proposed to explain the recent WILSON ET AL. REGIONAL CH4 SOURCES IN THE AMAZON BASIN 1 Global Biogeochemical Cycles 10.1002/2015GB005300 stabilization and subsequent regrowth of the atmospheric CH4 budget, none of them has yet been proven conclusively. Proposed explanations of the recent stalling have included a decrease in emissions from fossil fuels [e.g., Aydin et al., 2011; Simpson et al., 2012; Kirschke et al., 2013], stabilization of both fossil fuel and microbial sources [Levin et al., 2012], reductions in emissions from rice paddies due to improvements in prac- tices [Kai et al., 2011], and variations in the magnitude of the atmospheric sink of CH4 [Rigby et al., 2008; Montzka et al., 2011]. However, without better evaluation of CH4 surface fluxes, particularly from tropical regions, it is difficult to properly attribute changes in the atmospheric growth rate to variations in sources and sinks. The total global emission of methane into the atmosphere from wetland sources depends both on global wetland extent and on methane emission rate, although neither of these factors is currently well constrained [Ringeval et al., 2010; Melton et al., 2013]. The tropical regions make up the majority (50 – 60%) of the total glo- bal natural wetland CH4 source [e.g., Cao et al., 1996; Bloom et al., 2010, 2012], and inundated wetland regions in the Amazon Basin of South America, which covers an area of approximately 6,000,000 km2 [Poulter et al., 2010], have been estimated to contribute significantly (24%) [Bloom et al., 2012]. Previous estimates of CH4 emissions from the basin range from 4 to 92 Tg(CH4)/yr [do Carmo et al., 2006; Kirschke et al., 2013], but until recently, a scarcity of in situ observations of atmospheric CH4 concentrations in this region has restricted the possibility of thorough evaluation of these emission estimates. There have been previous attempts to use inverse modeling methods along with satellite observations of atmospheric CH4 concentrations in order to constrain emissions from the basin. However, due to limitations such as retrieval errors, the limited accuracy and coverage of remotely sensed CH4 over the region, and a lack of in situ measurements, these studies obtained artificially large total emissions [Frankenberg et al., 2006, 2008] or relatively small uncertainty reductions compared to better-observed regions [e.g., Bergamaschi et al., 2009; Fraser et al., 2013]. In 2010, to remedy this situation, we initiated regular measurements of various atmospheric species, includ- ing CH4, carbon monoxide (CO), carbon dioxide (CO2), and sulphur hexafluoride (SF6), at four sites within the Amazon Basin [Gatti et al., 2014]. On an approximately biweekly basis, vertical profiles of these species are measured over four locations at Santarem (SAN), Rio Branco (RBA), Tabatinga (TAB), and Alta Floresta (ALF), all of which are in the Brazilian part of the Amazon Basin (see Figure 1). These vertical profiles extend from just above the forest canopy to approximately 4.4 km above sea level, and the locations were selected to be sensitive to fluxes from the entire basin. This study uses these new observations of CH4, CO, and SF6 along with a chemical transport model, TOMCAT [Chipperfield, 2006], and two wetland emission models [Clark et al., 2011; Bloom et al., 2012] to reduce the uncertainty regarding methane emissions from the Amazon Basin during 2010 and 2011 and to assess the interannual uncertainty. We link differences between observed and simulated SF6 concentrations at the Amazonian measurement sites to errors in the model latitudinal transport. Quantifying this model transport error then allows us to update the simulated background CH4 concentrations at each of the sites. The use of tagged tracers
Details
-
File Typepdf
-
Upload Time-
-
Content LanguagesEnglish
-
Upload UserAnonymous/Not logged-in
-
File Pages21 Page
-
File Size-