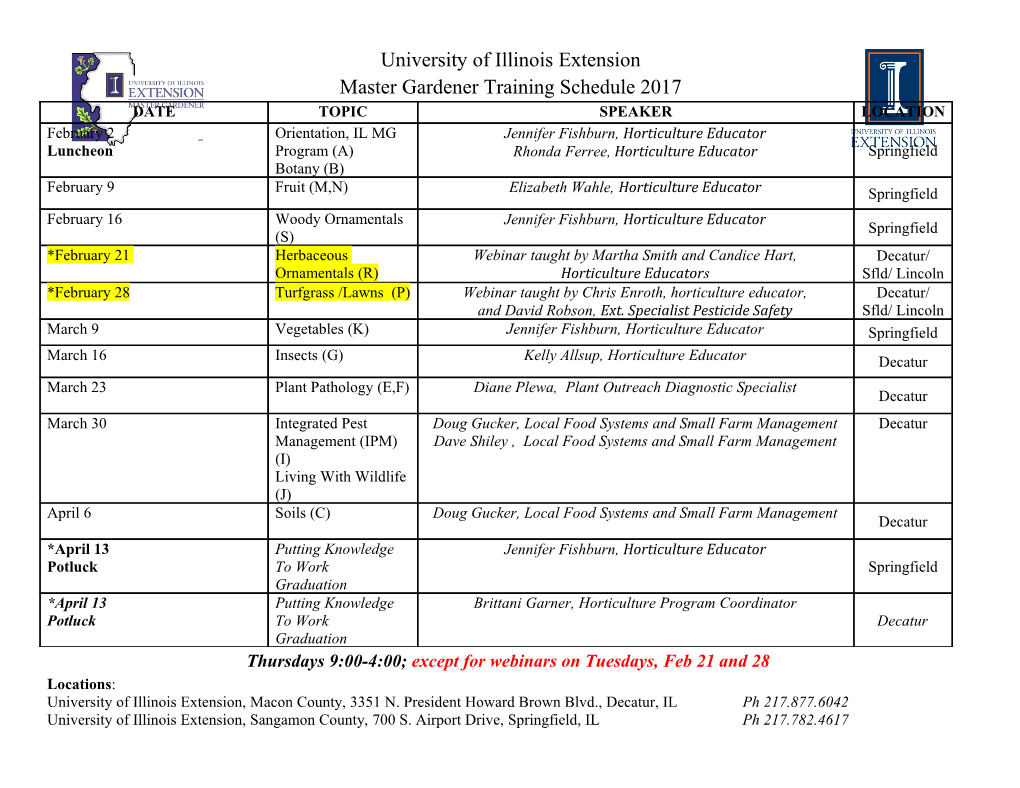
978 LPSC XIX PRELIMINARY ANALYTICAL ELECTRON MICROSCOPE (AEM) RESULTS OF NATURAL "GRAPHITE" SHOCKED AT 20.7, 31.4, 40.4 AND 59.1 GPa. Frans J. M. Rietmeijer, Department of Geology, University of New Mexico, Albuquerque NM 87131, USA. Elemental carbons, including graphite and hexagonal and cubic diamonds, occur in fine- grained chondritic materials1-3. Although shock metamorphism may be an important phase in the evolution of chondritic materials and interstellar dust4, detailed mineralogical data on shock -metamorphism of natural crystalline elemental carbons ("graphites") are rare. Laboratory shock experiments of elemetal carbons have produced complex shock-metamorphic assernblag~es~-~in support of a "hot spotW/"weakspot" response5 to the dynamic pressure/temperature regimes of a shock event. The scheduled deployment of a Space Station Cosmic Dust Collection ~acilit~~has prompted a study of Sri Lanka "graphites"g shocked at various peak pressures using a powder gun at NASA Johnson Space Center. The response of minerals to the energy of impact onto a collector must be determined in order to evaluate the type of iriformation that may survive an impact event. Ultramicrotomed thin sections of unshocked and shocked "graphites" were analysed using a JEOL 2000FX AEM operating at an accelerating voltage of 200kV and with an attached Tracor-Northern TN5500 energy dispersive spectrometer (EDS). The absence of characteristic X-ray signals for elements with atomic number > 11. was used for carbon grain selection. Selected area electron diffraction (SAED) data have a relative error of <lO/o. RESULTS. Carbon grains form irregular angular flakes that typically show polycrystalline SAED patterns (see data in TABLE 1) upon which a carbon single crystal pattern may be superimposed in sample 20.7. All samples, including unshocked "graphite", contain irregularly distributed twins, which can be clearly seen in dark field (DF) images ilsing the chaoite (201) reflection (d= 0.374nm). In all polycrystalline SAEB patterns, interplanar spacings between d= 0.238 - 0.224nm define a zone rather than distinct rings for these reflections. DF imaging using reflections from this zone shows (1) angular sub-grains (>200nm, in diameter) with low-angle boundaries and dislocation networks in all shocked samples, (2) hexagonal dislocation sub- structures (< -100nm, in diameter) in sample 59.1 and (3) parallel and rotational Moire patterns. Polycrystalline and single crystal chaoite Is the only elemental carbon phase although the inter- planar spacings, d= 0.314, 0.328, 0.369, 0.518, 0.539 and 0.587nm, do not fit chaoite [TABLE 11. In addition, interplanar spacings, d= 0.230 - 0.238nm, in polycrystalline and single crystal SAED patterns do not fit elemental carbons. It has been suggested that natural "graphites"lo'll contain linear carbon polytypes, or carbynes, which include chaoite12.' Carbynes have been pro- duced experimentally by heating hexagonal graphite or by raphitisation of diamond1'. Indeed, interplanar spacings d= 0.328nm n 0.369nm fit a-carbynel'. This result appears to violate the maxina that increased shock energy favours the stability of high-density phases. It is not clear why a-carbyne (p= 2.68 g.cmV3) should persist up to -60 GPa in the presence of chaoite (p= 3.43 g.cm-3). Most interplanar spacings [TABLE 11 that do not fit chaoite or a-carbyne are consistent with Sic. The presence of one or more SiC phase is puzzling since EDS analyses do not show evidence for Si (at the > 2 wt% level) in selected carbon grains. However, a cubic Sic phase (cell side = 1.23nm) is formed during experimental graphitisation of diamonds containing only 0.04-1.0 wt% SiO l4,l6.Also, in carbon vapor deposition experiments, carbon-VI, another form of carbynell, is Formed along with chaoite16. This carbon-VI is considered "an impurity- modified form of chaoite" containing an insi nificant amount of Al, Si, Ca, Cr or ~e~~ . Yet, the electron diffraction data for carbon YI1' are similar to those reported for SiC formed in graphitised diamonds14115.Indeed, interplanar spacings, d= 0.314, 0.369 and 0.587nm [TABLE 11 are consistent with this experimentally produced sic14-16. Thus, at least one Sic phase could already be present in the unshocked "graphite"g but the amount of Si is below the EDS detect- ion limit. As it is unlikely that Sic with -70wt% SiOa remains unnoticed during EDS analysis, it appears that very low silica contents in the C - Si binary system may result in conlplex mineralogies. Although Moire patterns in shocked "grapllites" are consistent with thin layers of chaoite and Sic, I suggest the possibility of chaoite polytypes that may form via shock-pressure induced dislocation reactions5, in order to account for all the diffraction data in TABLE 1. DISCUSSION. The lack of disc-shaped carbon grains indicates that melting did not although pseudomelting17 may go unnoticed. The presence of subgrains and sub-structures O Lunar and Planetary Institute Provided by the NASA Astrophysics Data System Shocked Graphite LPSC XIX 979 Rietmeijer, F. J. M. shows that a significant amount of recovery occurred in shocked "graphites". To test a model of chaoite polytypes to explain the chemical and SAED data for shocked "graphites", electron diffraction spacings for chaoite polyt pes with a= 0.895nm [=a ] and c= MC have been calculated using the RAD programsY8. In the model. C = l!&%%rn [=cchaoite]and M the multiplicity of a series of chaoite unit cells. Indeed, chaoite polytypes [TABLE 21 account for all data in TABLE I and suggest a rather complex shock-metamorphic mineralogy for nominally carbon phases. However. I conjecture the possibility of very low impurity metal contents in C - Metal binary systems (e.g. C-Si) may cause the formation of Magneli structures and long-periodic superstructures in natural "graphites" and which underscores the need for carefully monitored experiments. Fred Horc (NASAIJSC) provided the samples. This work is supported by NASA grant NAG 9-160. - .- .. .- - - -. .. -- -- - -- -. .. -- - -. .. UNS~IOCL~I SHOCKP.D-SAKPLES 20.7 11.6 40.4 REFERENCES. 1. Ftietmeijer FJM (1988) Icarus, in press; 2. Blake D et al. (1987) Nature, in press; 3. Rietmeijer FJM 18.21 10.35 & Mackinnon IDR (1987) Nature 326, 162; 4. Tielens 8.95 8.00 AGGM et al. (1987) Ap. J. 319, L109; 5. Kleiman J et al. 7.76 5.87 5.74 (1984) J. Appl. Phys. 66, 1440; 6. Sekine T et al. (1987) J. 5.31 5.33 5.18 5.125 Mat. Sci. 22, 3615; 7. Bundy FP (1980) J. Geophys. Res. 4.91 4.745 85(B12), 6930; 8. Horz F (ed) (1986) LPI Tech. Rpt 86-05, 102p; 9. Heyrnann D (1987) LPSC XVIII, 419; 10. Whittaker AG (1979) Carbon 17, 21; 11. Heimann RB et al. (1984) Carbon 22, 147; 12 Heirnann RB et al. (1983) Nature 306, 164; 13. Kasatochkin VI et al. (1973) Carbon 11, 70; 14. Seal M (1960) Nature 185, 522; 15. Raal FA (1960) Nature 185, 523; 16. Whittaker AG & Wolten GM (1972) Science 178, 54; 17. Bundy FP et al. (1973) in Chem. Phys. Carbon, 10 (PL Walker & PA Thrower, Eds) 213-264 (M Dekker, Inc., NY); 18. Cam MJ & Chambers WF (1984) J. Microsc. 134, 55. unshocked 'graphite" ch>oite(p) sample 20.7 chaoite(p;s) chaoite2(p) chaoite4(p;s) sample 31.4 chaoite(p) chaoite(3Xp) sample 40.4 chaoite(p) chaoile2(p) sample 59.1 chaoite(p) 1.15 1.11 1.12 1.10 1.10 TABLE II: Polycrystalline (p) and single crystal (s) chaoite and chaoite poly- TADLE r: EMmn difrnclion lalpbrisr (d) apsinn (A) Iw uoshackcd and rhoskcd wl~~tnlline':nehia'. hmp'* &lor tha Ihock prrnure (Gh)'Or types (MP2, 3 & 4) in experimentally-sh~kednatural 'graphites". txb ''rapkite. nrnpla. O Lunar and Planetary Institute Provided by the NASA Astrophysics Data System .
Details
-
File Typepdf
-
Upload Time-
-
Content LanguagesEnglish
-
Upload UserAnonymous/Not logged-in
-
File Pages2 Page
-
File Size-