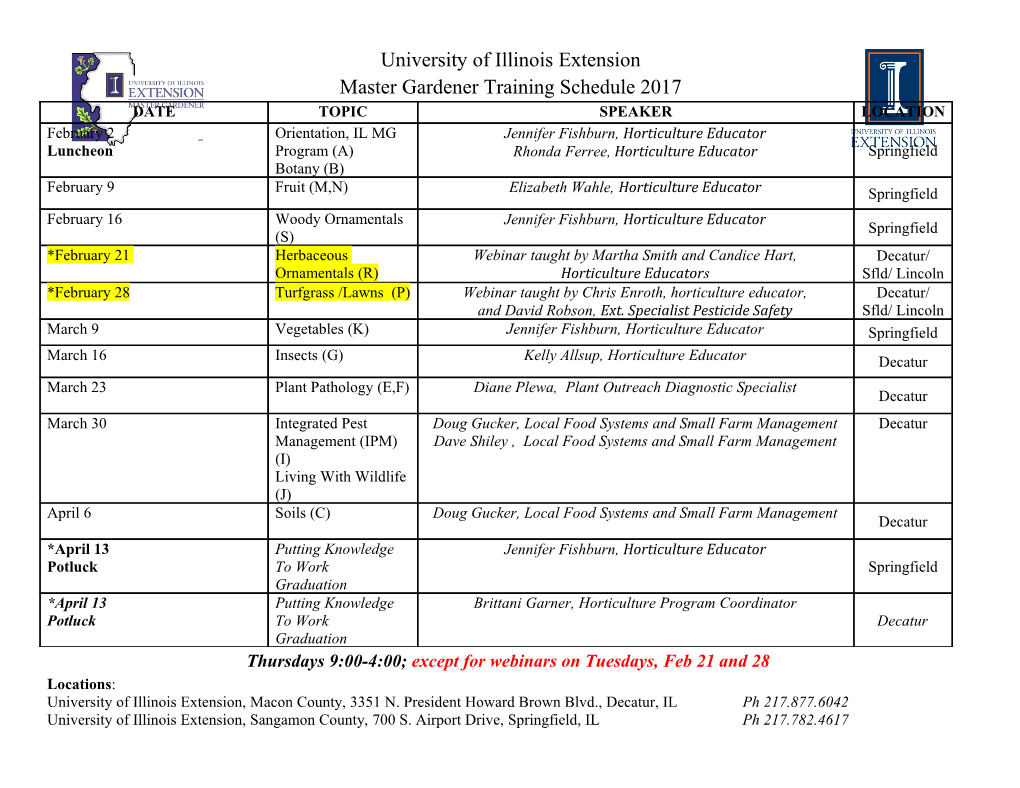
Chapter 16 NITROGEN 16-1 Introduction The nitrogen atom (ls22s22Px2py2pz) can complete its valence shell in the fol­ lowing ways: 3 1. Electron gain to form the nitride ion N -; this ion is found only in saltlike nitrides of the most electropositive metals. 2. Formation of electron-pair bonds: (a) single bonds, as in NH3 , or (b) multiple bonds, as in :N=N:, -N=N-, or N02 • 2 3. Formation of electron-pair bonds with electron gain, as in NH2or NH -. 4. Formation of electron-pair bonds with electron loss, as in the tetrahedral ammonium and substituted ammonium ions, [NR4 ]+. The following structural types (recall Chapter 3) are common among those compounds of nitrogen having covalent bonds: AB 4 (as in tetrahedral NR:); AB 3E (as in pyramidal NR3 ); AB 2E2 (as in bent NR2); AB3 (as in planar NOg); AB 2E (as in bent R2 C=N-OH); and ABE (N2 ). There are a few stable species in which, formally, the nitrogen valence shell is incomplete. Nitroxides, R2N=Q, NO, and N02 are the best examples; these have unpaired electrons and are paramagnetic. Three-Covalent Nitrogen The molecules NR3 are pyramidal; the bonding is best considered as involving sp3 hybrid orbitals so that the lone pair occupies the fourth position. There are three points to note: 1. As a result of the nonbonding electron pair, all NR3 compounds behave as Lewis bases and they give donor-acceptor complexes with Lewis acids, for example, F3B:N(CH3h, and they act as ligands toward transition metal ions as in, for example, [Co(NH3 )6]3+. 2. Pyramidal molecules (NRR'R") should be chiral. Optical isomers cannot be isolated, however, because such molecules very rapidly undergo a mo­ tion known as inversion in which the N atom oscillates through the plane of the three R groups, much as an umbrella can turn inside out (Fig. 16-1). The energy barrier for this process is only about 24 kJ mol-I. 3. There are a very few cases where three-covalent nitrogen is planar; in these cases multiple bonding is involved as we discussed for N (SiMe3 ) 3' in 399 400 Chapter 16 / Nitrogen Q' N R" \:';R" ,'I ",\: ~ R::_-- R' R-"I -R.' 'II N (j Figure 16·1 Diagram illustrating the inversion of NHg • Section 15-1. The N-centered triangular metal complexes such as [NIr3(S04)6(H20)3]4- are similar. N-N Single-Bond Energy The N-N single bond is quite weak. If we compare the single-bond energies: HO-OH F-F Units 350 160 140 150 kJ mol-1 it is clear that there is a profound drop between C and N. This difference is prob­ ably attributable to the effects of repulsion between nonbonding lone pairs. The result is that, unlike carbon, nitrogen has little tendency to catenation. Multiple Bonds The propensity of nitrogen, like carbon, to form jJTt-jJTt multiple bonds is a fea­ ture that distinguishes it from phosphorus and the other Group VB(l5) ele­ ments. Thus nitrogen as the element is dinitrogen (N2), with a very high bond strength and a short internuclear distance (1.094 A), whereas phosphorus forms p4 molecules or infinite layer structures in which there are only single bonds (Section 8-5). Where a nitrogen atom forms one single and one double bond, nonlinear molecules result, as shown in Structures 16-1 to 16-IV. R1 R2 "'/ C II N. /. HO 16-1 Each nitrogen atom in these structures is of the AB2E type, uses Sp2 hybrid or­ bitals, and forms a 1t bond using the unhybridized 2p orbital. 16-3 Nitrides 401 In the oxo anions NO; (AB2E) and N0:3 (AB3), there are multiple bonds that may be formulated in either resonance or MO terms, as discussed in Chapter 3. 16-2 Occurrence and Properties of the Element Nitrogen occurs in nature mainly as dinitrogen, N2 (bp 77.3 K), which comprises 78% by volume of the earth's atmosphere. The isotopes 14N and 15N have an ab­ solute ratio 14N/15N = 272.0. Compounds enriched in 15N are used in tracer stud­ ies. The heat of dissociation of N2 is extremely large. 1 120 t:.H= 944.7 kJ mol- K 25 •C = 10- (16-2.1) The great strength of the N-N bond is principally responsible for the chemical inertness of N2 and for the fact that most simple nitrogen compounds are en­ dothermic even though they may contain strong bonds. Dinitrogen is notably unreactive in comparison with isoelectronic, triply bonded systems such as X-C-C-X, :C=O:, X-C-N:, and X-N=C:. Both -C C- and -C-N groups can act as donors by using their n; electrons, whereas N2 does not. It can, however, form complexes similar to those formed by CO, although to a much more limited extent, in which there are Mf-N= : and Mf-C=O: configura­ tions (Chapter 28). Nitrogen is obtained by liquefaction and fractionation of air. It usually con­ tains some argon and, depending on the quality, upwards of about 30 ppm of oxygen. Spectroscopically pure N2 is made by thermal decomposition of sodium or barium azide. (16-2.2) The only reactions of 2 at room temperature are with metallic Li to give Li3N, with certain transition metal complexes, and with nitrogen fixing bacteria. These nitrogen fixing bacteria are either free living or symbiotic on the root nod­ ules of clover, peas, beans, and the like. The mechanism by which these bacteria fix N2 is unknown. At elevated temperatures nitrogen becomes more reactive, especially when catalyzed. Typical reactions are 3 2 N2(g) + 3 H 2(g) = 2 H3(g) K 25 •C = 10 atm- (16-2.3) 31 N2(g) + 02(g) = 2 NO(g) K 25 •C = 5 X 10- (16-2.4) N2(g) + 3 Mg(s) = Mg3N2(s) (16-2.5) N2(g) + CaC2(s) = C(s) + CaNCN(s) (16-2.6) 16-3 Nitrides Nitrides of electropositive metals have structures with discrete nitrogen atoms and can be regarded as ionic, for example, (Ca2+)3(N3-h and (Li+)3N3-. Their ready hydrolysis to ammonia and the metal hydroxides is consistent with this. 402 Chapter 16 / Nitrogen Such nitrides are prepared by direct interaction or by loss of ammonia from amides on heating, for example, (16-3.1 ) Transition metal nitrides are often nonstoichiometric and have nitrogen atoms in the interstices of close-packed arrays of metal atoms. Like the similar carbides or borides they are hard, chemically inert, high melting, and electrically conducting. There are numerous covalent nitrides (BN, S4N4' PSN 5, etc.), and their prop­ erties vary greatly depending on the element with which nitrogen is combined. These are, therefore, discussed more fully under the appropriate element. 16-4 Nitrogen Hydrides Ammonia Ammonia (NHs) is formed by the action of a base on an ammonium salt. (16-4.1) Industrially, ammonia is made by the Haber process in which the reaction !1H = -46 kJ mol-1 (16-4.2) 2 • K 25 C = lOS atm- is carried out at 400-500 °c and pressures of 102-10s atm in the presence of a catalyst. Although the equilibrium is most favorable at low temperature, even with the best catalysts, elevated temperatures are required to obtain a satisfactory rate. The best catalyst is a-iron containing some oxide to widen the lattice and enlarge the active interface. Ammonia is a colorless, pungent gas (bp -33.35 0C). The liquid has a large heat of evaporation (1.37 kJ g-l at the boiling point) and can be handled in or­ dinary laboratory equipment. Liquid NHs resembles water in its physical behav­ ior, being highly associated via strong hydrogen bonding. Its dielectric constant (-22 at -34°C; cf. 81 for H 20 at 25°C) is sufficiently high to make it a fair ion­ izing solvent. Its self-ionization has been discussed previously (Section 7-3). Liquid NHs has lower reactivity than H 20 toward electropositive metals and dissolves many of them (Section 10-3). Because NHs(f) has a much lower dielectric constant than water, it is a bet­ ter solvent for organic compounds but generally a poorer one for ionic inor­ ganic compounds. Exceptions occur when complexing by NHs is superior to that by water. Thus AgI is exceedingly insoluble in water but very soluble in NHs. Primary solvation numbers of cations in NHs appear similar to those in H 20, for 2 s example, 5.0 ± 0.2 and 6.0 ± 0.5 for Mg + and Al +, respectively. Ammonia burns in air: 228 K25 .C = 10 (16-4.3) 16-4 Nitrogen Hydrides 403 Reaction 16-4.3 is thermodynamically favored under normal conditions. However, at 750-900 °C, in the presence of a platinum or a platinum-rhodium catalyst, reaction ofammonia with oxygen can be made to give NO instead ofN2, as in Eq. 16-4.4: (16-4.4) thus affording a useful synthesis of NO. The latter reacts with an excess of O 2 to produce N02, and the mixed oxides can be absorbed in water to form nitric acid. 2 NO + O 2 -------'? 2 N02 (16-4.5) 3 O 2 + H 20 -------'? 2 HN03 + NO and so on (16-4.6) Thus the sequence in industrial utilization of atmospheric nitrogen is as follows: N H 2 NH O2 ) NO (16-4.7) 2 Haber ) 3 Ostwald process process Ammonia is extremely soluble in water. Although aqueous solutions are gen­ erally referred to as solutions of the weak base NH40H, called ammonium hy­ droxide, undissociated NH4 0H probably does not exist.
Details
-
File Typepdf
-
Upload Time-
-
Content LanguagesEnglish
-
Upload UserAnonymous/Not logged-in
-
File Pages17 Page
-
File Size-