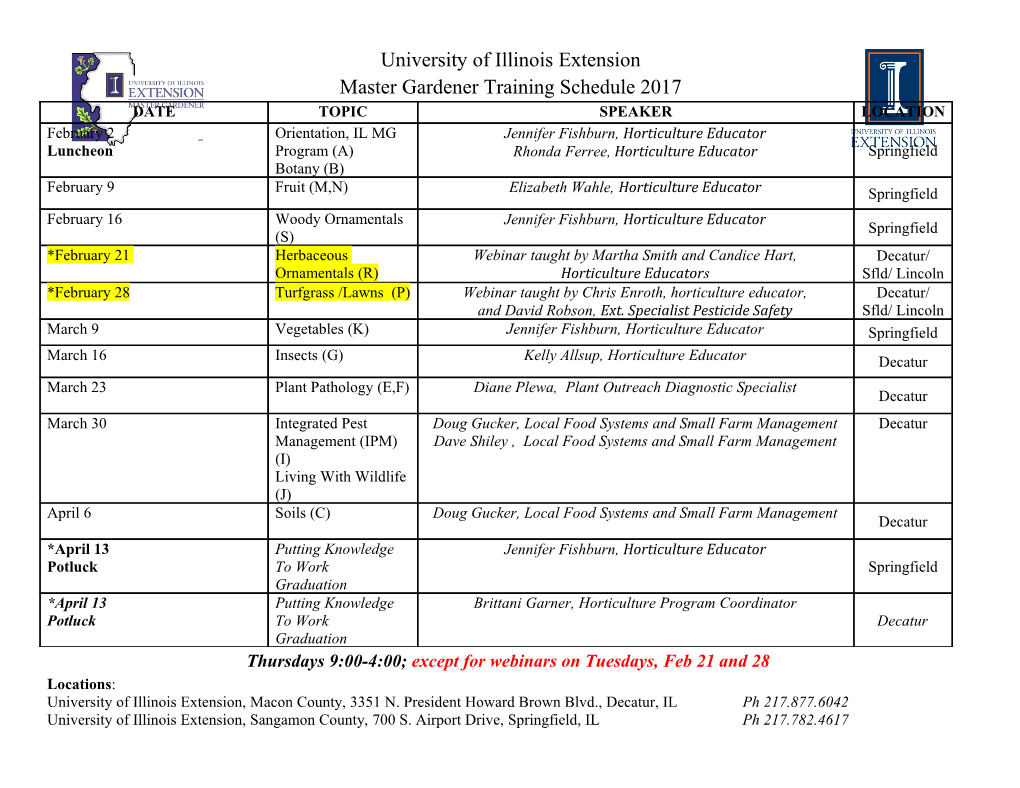
xÎ Þ`ÀÌ iÀ>Ê6iÌÊÕ`Ã]Ê-ViÕÃÊÞ`ÀÌ iÀ>Ê i«ÃÌÃ]Ê>`Ê Þ`ÀÌ iÀ>ÞÊÌiÀi`Ê-i`iÌÃÊÊ9iÜÃÌiÊ>i 7#0AT3HANKS )))1*\,ISA!-ORGAN1\ ,AURIE"ALISTRIERI2\*EFFREY#!LT 53'EOLOGICAL3URVEY $ENVER&EDERAL#ENTER #/ 53'EOLOGICAL3URVEY 5NIVERSITYOF7ASHINGTON3CHOOLOF/CEANOGRAPHY 3EATTLE $EPARTMENTOF'EOLOGICAL3CIENCES 5NIVERSITYOF-ICHIGAN !NN!RBOR #ORRESPONDING!UTHOR 53'EOLOGICAL3URVEY $ENVER&EDERAL#ENTER $ENVER #/ 0HONE%MAILPSHANKS USGSGOV x{ "/ ,Ê ""9Ê Ê " -/,9Ê Ê9 "7-/" Ê /" Ê*, -/, / 3TABLEISOTOPICδ$ANDδ/ DATAINDICATEABOUTTOTALEVAPORATIVECONCENTRATIONHASOCCURREDIN9ELLOWSTONE,AKE YET LAKEWATERSAREENRICHEDINDISSOLVED!S " #L #S 'E ,I -O 3B AND7BYATLEASTANORDER OF MAGNITUDERELATIVETOTHE mOW WEIGHTEDCOMPOSITIONOFINmOWINGSTREAMS7ECONCLUDETHATLAKEWATERISAMIXTUREOFINmOWINGSURFACEWATERAND HYDROTHERMALSOURCEmUIDTHATISSTRONGLYENRICHEDIN#LANDOTHERELEMENTS7EESTIMATETHAT^OFTHETOTALHYDROTHERMAL mUXIN9ELLOWSTONE.ATIONAL0ARK9.0 OCCURSIN9ELLOWSTONE,AKE'EOCHEMICALANDMINERALOGICALSTUDIESOFHYDROTHERMAL DEPOSITSANDHYDROTHERMALLYALTEREDLAKESEDIMENTSVENTMUDS FROMTHEACTIVEORRECENTLYACTIVEVENTSITESONTHEmOOROF 9ELLOWSTONE,AKEINDICATETHATTHEIRFORMATIONISDUETOHYDROTHERMALmUIDQUENCHINGDURINGmOWTHROUGHSHALLOWCONDUITS ORTOMIXINGUPONEGRESSINTOCOLDBOTTOMWATERS3ILICEOUSPRECIPITATESFORMCONDUITSWITHINTHEUPPERMOSTSEDIMENTS TABULAR DEPOSITSALONGSEDIMENTARYLAYERS ANDSPIRESUPTOMTALL4HESEDEPOSITSAREENRICHEDIN!S #S (G -O 3B 4LAND73PIRES VENTDEPOSITS ANDCONDUITSCONTAINlLAMENTOUSMICROSTRUCTURESTHATPROBABLYREPRESENTSILICIlEDBACTERIA0ARTLYRECRYSTALLIZED ANDSILICIlEDDIATOMSAREABUNDANTINDEPOSITSBELOWTHESEDIMENT WATERINTERFACE6ENTMUDSANDSOMEOUTERCONDUITWALLS SHOWPERVASIVELEACHINGOFSILICA WHICHEXPLAINSTHEOCCURRENCEOFMOSTSUBLACUSTRINEVENTSINCRATERS3YSTEMATICSOFδ$AND #LVARIATIONS ASWELLASSILICAANDCATIONGEOTHERMOMETRYFORHYDROTHERMALmUIDS SUGGESTTHATASCENDINGmUIDSBOILDUETO DEPRESSURIZATIONTOATEMPERATUREOF^O#ANDTHENMIXWITHPOREWATERSPRIORTOVENTINGONTHELAKEBOTTOM$EPOSITIONAL TEMPERATURESFORSUBLACUSTRINESILICADEPOSITS CALCULATEDUSINGOXYGENISOTOPEFRACTIONATION RANGEFROMO#TOO#4HE AMORPHOUSSILICA SATURATEDVENTmUIDSPRECIPITATESILICATOFORMSPIRESORCONDUITSLARGELYBYCONDUCTIVECOOLING"ACTERIAL ACCUMULATIONSMAYHAVEINHIBITEDTHEMIXINGOFVENTmUIDSANDBOTTOMWATERS ANDPROVIDEDASITEFORSILICADEPOSITION iÞÊ7À`à >ÌiÀ>Ì Þ`ÀÌ iÀ> ÃV> ëÀià ÃÌ>LiÊÃÌ«ià ÌÀ>ViÊiiiÌà ÛiÌà Hydrothermal Vent Fluids, Deposits, and Sediments 55 1.0 INTRODUCTION (Wold et al. 1977), with a diameter of about 2.6 km, is the Yellowstone National Park (YNP) is a region of recent largest known crater of this type in the world (Browne and and active tectonism and volcanism that is related to its Lawless 2001) and is still a center of intense sublacustrine current position over the Yellowstone hotspot (Pierce and hydrothermal activity (Figures 1A and 1C). Analogous Morgan 1992; Yuan and Dueker 2005). Large-volume, large hydrothermal explosion craters formed subaerially at high-silica rhyolite ignimbrites and lava flows have erupted Indian Pond, Turbid Lake, Duck Lake, and several other from overlapping and nested calderas in the Yellowstone locations in the Park (Muffler et al. 1971; Morgan et al. Plateau volcanic field. The last major eruption produced 1998; Pierce et al. 2002) the 0.64-Ma Yellowstone Caldera, which contains most of This report focuses on the geochemistry of Yellowstone the active hydrothermal basins in the Park. Seismic studies Lake water, the processes that control its composition, suggest that a zone of magma is present below the caldera and the supply of potentially toxic elements to the lake at a depth of about 5-6 km (Christiansen 2001; Smith and ecosystem by sublacustrine hydrothermal vents. Such Rubin 1994). Magmatic heat drives YNP’s abundant and information is critical for understanding the complex spectacular hydrothermal activity. relationships between geology and biological communities Isotopic and geochemical studies indicate that the in YNP. We also examine the hydrothermal processes impressive subaerial thermal basins in YNP result from at sublacustrine vent sites that alter lake sediments and the interaction between deeply recharged meteoric water produce lake-bottom siliceous deposits. and a magma chamber in the upper crust beneath the Yellowstone Caldera (Fournier 1999). A deeply circulating, 2.0 METHODS hot (360oC), chloride-enriched fluid reservoir (Fournier 1989; Rye and Truesdell 1993, and in press) is thought During August and September of 1998, samples of to feed most thermal fields and geyser basins in the park. surface water were collected and flow measurements were Temperature and compositional variations between hot made at 44 of the 141 streams that enter Yellowstone springs are due to a series of intermediate reservoirs that Lake; these were the streams with highest flow. In July alter fluids by conductive cooling to surrounding rocks, 1999, sampling and flow measurements were redone for 21 of the 44 streams—those established as significant steam separation and H2S oxidation, and mixing and dilution of ascending hydrothermal fluids with colder contributors to the lake due to their high flow and amount meteoric water (Truesdell et al. 1977; Fournier 1989, and of metal loading in the water. The resulting data allow this volume). Water-rock interaction, magma degassing, calculation of flow-weighted influx for comparison with and mineral precipitation also contribute to the diverse outflow at the U.S. Geological Survey (USGS) gauge of geochemical compositions of YNP hot springs. the Yellowstone River at its outlet from the lake (station 06186500; http://waterdata.usgs.gov/mt/nwis/). Samples Multiple geologic forces—volcanic, hydrothermal, and from different depths in the water column of Yellowstone glacial—have shaped the Yellowstone Lake basin Lake were collected in Southeast Arm, West Thumb, and (Morgan et al. 2003; Morgan and Shanks, this volume; Mary Bay in 1997 and 1998, and near Stevenson Island Figure 1, next page). The northern portion of the lake in 1998, using trace-metal-clean hydro bottles and non- and West Thumb basin are within the Yellowstone contaminating hydro cable. Caldera (Figure 1A) and host numerous sublacustrine hydrothermal vent sites. In addition, large sublacustrine Hydrothermal vent waters and siliceous hydrothermal hydrothermal explosion events formed Mary Bay crater, deposits and sediments were collected from the lake Elliott’s crater, Evil Twin Crater in West Thumb basin, bottom with a tethered remotely operated vehicle (ROV) and the unnamed crater south of Frank Island (Morgan designed and piloted by David Lovalvo of Eastern et al. 2003). The Mary Bay hydrothermal explosion crater Oceanics, Inc. Water samples were collected in large plastic 56 GEOTHERMAL BIOLOGY AND GEOCHEMISTRY IN YELLOWSTONE NATIONAL PARK A. Yellowstone Lake PELICAN YELLOWSTONE MaryBay RIVER OUTLET CR. crater 44°32'30"N Bridge Elliott's Steamboat Figure 1. Bathymetric maps Bay BRIDGE crater Point CR. of: A. Yellowstone Lake, B. West Thumb showing locations of Sedge Stevenson 44°30'0"N Island Bay hydrothermal sites, and C. Northern CUB Yellowstone Lake showing locations CR. ARNICA of hydrothermal sites. CR. CLEAR CR. 44°27'30"N West Thumb Dot Island MEADOW Frank CR. Island 44°25'0"N COLUMBINE CR. SOLUTION CR. in arg M 44°22'30"N ra Southeast e Arm d FLAT l MOUNTAIN a STREAM C 44°20'0"N South Arm YELLOWSTONE RIVER INLET CHIPMUNK GROUSE CR. 44°17'30"N CR. TRAIL CR. 110°35'0"W 110°30'0"W 110°25'0"W 110°20'0"W 110°15'0"W 0 km 3 B. West Thumb C. Northern Yellowstone Lake Mary Bay deep hole vents and 44°35'N Indian hot core Pond Bridge Bay spires inflated Storm Steamboat plain Point Point vents Evil Twin vents crater and vents Elliott's Duck Crater Sedge Lake cutthroat West Thumb 44°25'N Bay jacuzzi deep vent Stevenson vents West (hot core) 44°30'N Thumb Island vents Geyser Basin W. Thumb off Grant Marina 110°25'W 110°20'W 110°35'W 110°30'W Hydrothermal Vent Fluids, Deposits, and Sediments 57 syringes attached to sampling tubes fitted, along with a temperature probe, to an articulated telescoping sampling water sampling arm. The ROV also was equipped with a motorized scoop water arm for collecting solid and sediment samples. Some additional sampling syringe sediment samples were collected by gravity coring, and spire samples were collected by the National Park Service pan and Yellowstone dive team. A typical configuration of the tilt camera submersible is shown in Figure 2. solid Alkalinity, pH, and temperature of water samples were sampling scoop measured in the field. Laboratory analyses for 40 major and minor elements were obtained by inductively coupled University of Minnesota plasma-mass spectrometry (ICP-MS); Hg by cold vapor in situ H2-H2S-pH-T probe atomic fluorescence; anions by ion chromatograph; and Figure 2. Photo of the Eastern Oceanics, Inc. remotely δ18O, δD, and δ34S by isotope ratio mass spectrometry operated vehicle (ROV) for submersible studies in calibrated to Vienna Standard Mean Ocean Water Yellowstone Lake. (VSMOW) or Canyon Diablo troilite (CDT). Analytical (X-ray fluorescence and ICP-MS) and isotopic analyses in errors based on replicate analyses of standards are <0.05 the USGS laboratories in Denver, CO. Silicate samples for per mil for δ18O , ~1.0 per mil for δD, and <0.2 per mil H2O oxygen isotope analysis were reacted with BrF following for δ34S. 5 the Clayton and Mayeda (1963) technique. Sulfur-bearing Minerals were characterized by X-ray diffraction (XRD), phases were analyzed for δ34S using an automated elemental scanning electron microscopy (SEM), and by chemical analyzer interfaced to an isotope ratio mass
Details
-
File Typepdf
-
Upload Time-
-
Content LanguagesEnglish
-
Upload UserAnonymous/Not logged-in
-
File Pages20 Page
-
File Size-