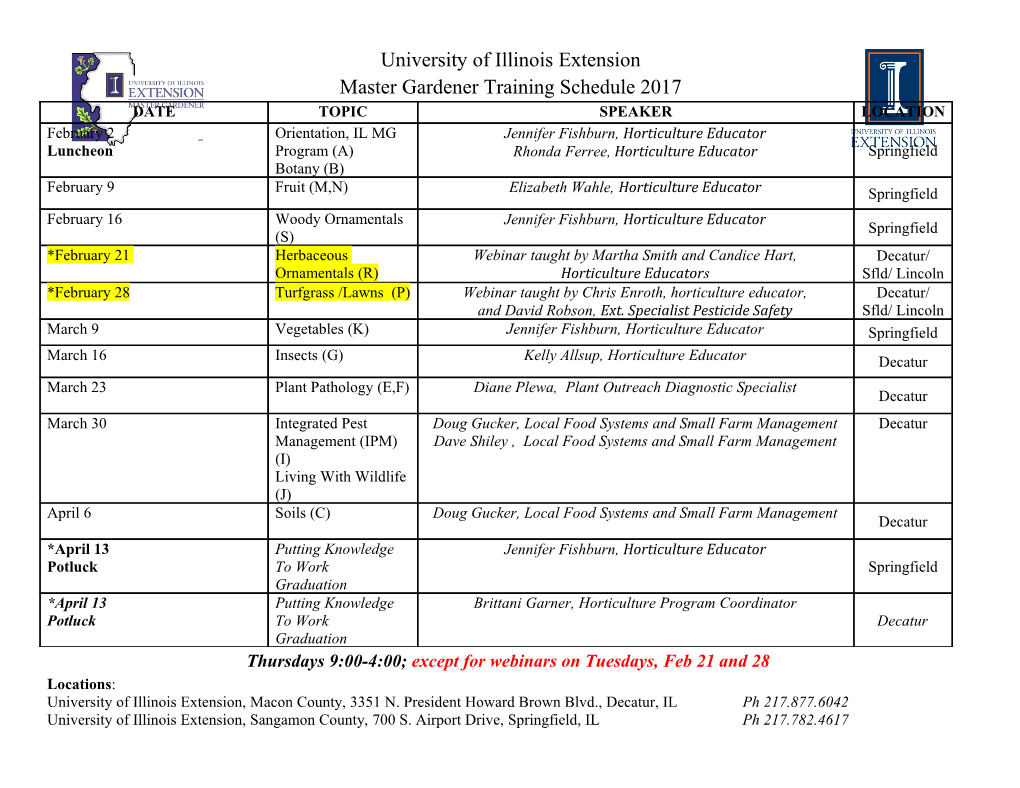
Biogeosciences, 11, 6357–6375, 2014 www.biogeosciences.net/11/6357/2014/ doi:10.5194/bg-11-6357-2014 © Author(s) 2014. CC Attribution 3.0 License. Impact of droughts on the carbon cycle in European vegetation: a probabilistic risk analysis using six vegetation models M. Van Oijen1,2, J. Balkovi3, C. Beer4,5, D. R. Cameron1, P. Ciais6, W. Cramer7, T. Kato6,13, M. Kuhnert8, R. Martin9, R. Myneni10, A. Rammig11, S. Rolinski11, J.-F. Soussana9, K. Thonicke11, M. Van der Velde2, and L. Xu10,12 1Centre for Ecology & Hydrology, Edinburgh, EH26 0QB, UK 2Bioforsk, Saerheim, Norway 3International Institute for Applied Systems Analysis (IIASA), Schlossplatz 1, 2361 Laxenburg, Austria 4Max-Planck-Institute for Biogeochemistry, Jena, Germany 5Department of Applied Environmental Science (ITM), Bolin Centre for Climate Research, Stockholm University, Stockholm, Sweden 6Laboratoire des Sciences du Climat et de l’Environnement, CEA-CNRS-UVSQ, 91198 Gif-sur-Yvette, France 7Institut Méditerranéen de Biodiversité et d’Ecologie marine et continentale (IMBE), Aix Marseille Université, CNRS, IRD, Avignon Université, 13545 Aix-en-Provence, France 8University of Aberdeen, School of Biological Sciences, Aberdeen, UK 9INRA, Grassland Ecosystem Research, (UR 874), 63100 Clermont-Ferrand, France 10Department of Earth and Environment, Boston University, Boston, USA 11Potsdam Institute for Climate Impact Research (PIK), Telegrafenberg 31, 14473 Potsdam, Germany 12Institute of the Environment and Sustainability (IoES), University of California, Los Angeles, USA 13Research Faculty of Agriculture, Hokkaido University, Sapporo, 060-8589 Japan Correspondence to: M. Van Oijen ([email protected]) Received: 24 March 2014 – Published in Biogeosciences Discuss.: 5 June 2014 Revised: 25 September 2014 – Accepted: 23 October 2014 – Published: 26 November 2014 Abstract. We analyse how climate change may alter risks across Europe. For each grid cell, drought vulnerability and posed by droughts to carbon fluxes in European ecosys- risk are quantified for five seasonal variables: net primary tems. The approach follows a recently proposed framework and ecosystem productivity (NPP, NEP), heterotrophic respi- for risk analysis based on probability theory. In this ap- ration (Rh), soil water content and evapotranspiration. proach, risk is quantified as the product of hazard probabil- In this analysis, climate change leads to increased drought ity and ecosystem vulnerability. The probability of a drought risks for net primary productivity in the Mediterranean area: hazard is calculated here from the Standardized Precipita- five of the models estimate that risk will exceed 15 %. The tion–Evapotranspiration Index (SPEI). Vulnerability is cal- risks increase mainly because of greater drought probabil- culated from the response to drought simulated by process- ity; ecosystem vulnerability will increase to a lesser extent. based vegetation models. Because NPP will be affected more than Rh, future carbon We use six different models: three for generic vegeta- sequestration (NEP) will also be at risk predominantly in tion (JSBACH, LPJmL, ORCHIDEE) and three for spe- southern Europe, with risks exceeding 0.25 g C m−2 d−1 ac- cific ecosystems (Scots pine forests: BASFOR; winter wheat cording to most models, amounting to reductions in carbon fields: EPIC; grasslands: PASIM). The periods 1971–2000 sequestration of 20 to 80 %. and 2071–2100 are compared. Climate data are based on gridded observations and on output from the regional climate model REMO using the SRES A1B scenario. The risk anal- ysis is carried out for ∼ 18 000 grid cells of 0.25 × 0.25◦ Published by Copernicus Publications on behalf of the European Geosciences Union. 6358 M. Van Oijen et al.: Impact of droughts on the carbon cycle in European vegetation 1 Introduction difference between the actual average of the system variable and its value under continuously non-hazardous conditions. In the coming decades, climate change is expected to lead With these precise definitions, we achieve the desired de- to higher temperatures and to greater temporal variability composition: R D P .H / × V . All three terms are expected in precipitation and temperature in many parts of the world to change over time. Climate change, for example, directly (Seneviratne et al., 2006). Such changes will alter the fre- affects the probability of hazardous conditions, and changes quency, intensity and duration of droughts, with consequent in ecosystems alter their vulnerability. effects on the productivity and carbon balance of ecosys- We note that risk decomposition as defined in our PRA tems (Reichstein et al., 2013). Meanwhile, increasing CO2 framework is not equivalent to “fault tree analysis” (FTA) has been suggested to increase plant water use efficiency, as practised in engineering projects (Rausand, 2011). FTA and to save soil moisture by reducing stomatal conductance quantifies the failure probability of the different components (e.g. Drake et al., 1997; Morgan et al., 2004; Keenan et al., in a human-made system and this is modelled using discrete 2013), a mechanism which could partly alleviate the sensitiv- probability distributions. This approach is not suitable for our ity of plants and ecosystems to drought. How these changes purposes in ecology where response variables such as carbon will affect the vulnerability of vegetation to future drought fluxes are not binary: fluxes do not necessarily “fail” when and the associated risks has not yet been rigorously quanti- there is a drought but can change to any given degree. There- fied (Van Oijen et al., 2013b). fore our framework for risk analysis uses continuous prob- Risk analysis is applied in many fields, and terminology ability distributions and we define vulnerability as a func- is inconsistent (Brooks, 2003; Schneiderbauer and Ehrlich, tion of expectation values and not discrete probabilities. We 2004). Generally, “hazard”, “vulnerability” and “risk” are have not been able to find the PRA equations (given below in distinguished (DHA, 1992). “Hazard” refers to damaging Sect. 2.5.1 and more fully in Van Oijen et al., 2013b) in the conditions which may on occasion prevail. “Vulnerability” engineering literature, although of course there is conceptual refers to the sensitivity of the impacted system to those dam- similarity between the fields. aging conditions. “Risk” is commonly defined as an expecta- We apply the PRA framework here to the impact tion value for loss induced by the hazardous conditions. Al- of droughts on vegetation in Europe, both in recent though most work on risk analysis distinguishes three such (1971–2000) and future (2071–2100) years. Our focus is on terms, the definitions – in particular of vulnerability – are the responses of the major carbon fluxes between ecosys- generally too imprecise to place the terms in a mathematical tems and the atmosphere: net primary productivity (NPP), relationship to each other (Ionescu et al., 2009). Risk analy- heterotrophic respiration (Rh) and net ecosystem productiv- ses thus tend not to show how exactly risk should be decom- ity (NEP). The different carbon fluxes in ecosystems are posed into its two constituent terms, hazard and vulnerability. tightly linked to each other (e.g. Raich and Schlesinger, 1992; This hampers the use of risk analysis to ecosystems when we van Oijen et al., 2010), and we can therefore analyse how are interested not only in quantifying risk, or changes in risk, drought risks to carbon sequestration in the form of NEP but also in identifying the main causal factors. In the present result from risks to the other two carbon fluxes, given that study, we aim to disentangle two effects of climate change NEP D NPP − Rh. In addition, we examine the primary re- in a risk analysis framework. First, to what extent does cli- sponses of soil water content (SWC) and evapotranspiration mate change lead to increased probability of extreme, haz- (ET), giving five system variables in total. The water vari- ardous weather? Second, how does climate change affect the ables SWC and ET are included to determine whether risks vulnerability of ecosystems to such extreme conditions? For to NPP are mainly due to reductions in water availability or this, we require a quantitative method that allows risk to be in water use. We quantify how risks to these five variables un- decomposed. der future conditions differ from the present across Europe, Recently, we proposed a method for probabilistic risk and proceed by inspecting the two constituent terms of risk analysis (PRA) which is able to decompose the risk into two to pinpoint the main cause of change. constituent terms (Van Oijen et al., 2013b). The PRA is de- We define drought using the Standardized Precipita- signed to analyse the effect of any environmental variable tion–Evapotranspiration Index (SPEI, Vicente-Serrano et al., on any system variable of interest. An example could be the 2010). The SPEI is a localized measure of drought: it is based impact of drought on ecosystem carbon flux. The method for every site on the local long-term frequency distribution of allows for either variable to be derived from measurement precipitation minus potential evapotranspiration. The SPEI or from modelling. “Hazardous conditions” are defined as quantifies how rare a given difference of precipitation and those where the environmental variable is more extreme than potential evapotranspiration is, with respect to this frequency a given threshold, and their probability of occurrence is de- distribution. Because one aim of our analysis is to determine noted as P .H /. We define vulnerability (V ) as the difference in which parts of Europe climate change is expected to lead between the expectation values for the system variable un- to a change in the probability of drought (P .H /), we use the der non-hazardous and hazardous conditions. Risk (R) is de- same reference period for the SPEI calculations for both the fined, as is commonly done, as the expectation of loss: the present and future risk analysis, choosing 1901–2010 for this Biogeosciences, 11, 6357–6375, 2014 www.biogeosciences.net/11/6357/2014/ M.
Details
-
File Typepdf
-
Upload Time-
-
Content LanguagesEnglish
-
Upload UserAnonymous/Not logged-in
-
File Pages19 Page
-
File Size-