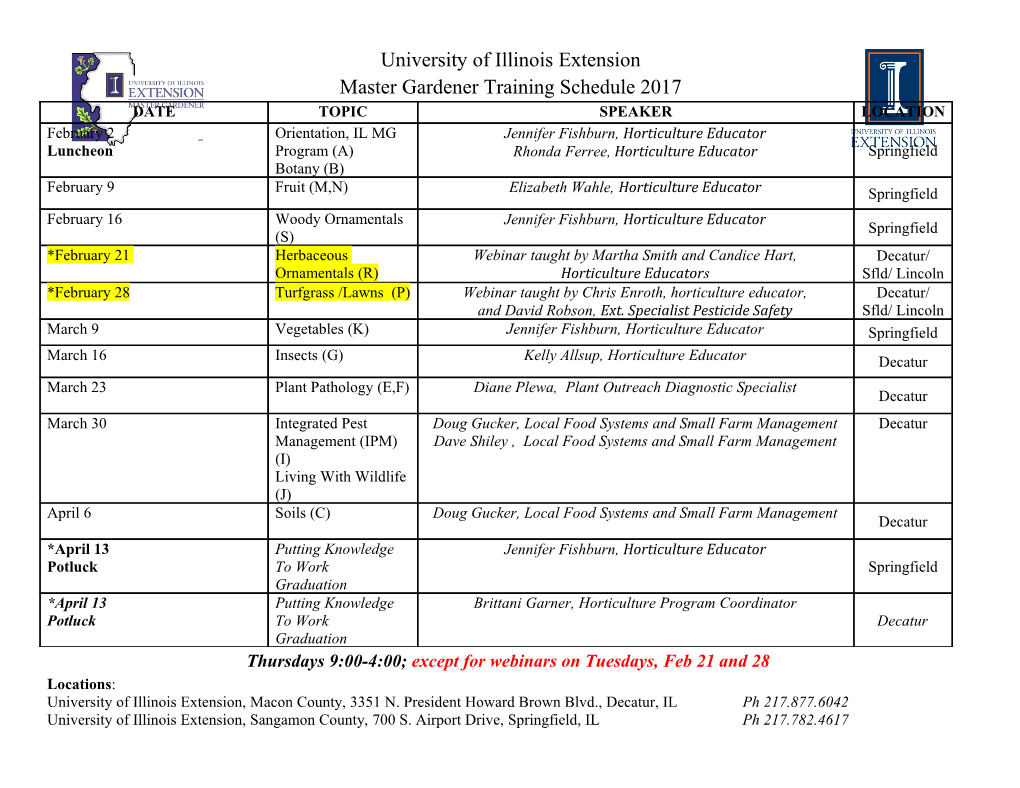
Chapter 31 Transcription 1. The role of RNA in protein synthesis 2. RNA polymerase 3. Control of transcription in eukaryotes 4. Posttranscriptional processing Three major classes of RNA All participate in protein synthesis: • Ribosomal RNA, rRNA 1. Transfer RNA, tRNA 2. Messenger RNA, mRNA All are synthesized from DNA by transcription Historically DNA found in cell nucleus, but RNA found in cytosol (1930), microscopy and cell fractionation Concentration of cytosolic RNA-Protein particles correlate with protein synthesis - is site of protein synthesis - was later identified as Ribosome In eukaryotes, DNA is never in association with protein synthesis (Ribosome) Incorporation of radiolabelled amino acids occurs in association with RNA-Protein particles Structure of DNA revealed possible copy mechanism Central Dogma DNA RNA Protein 1. The role of RNA in protein synthesis Studied by following enzyme induction Bacteria vary the synthesis of certain enzymes depending on environmental Conditions Enzyme induction occurs as consequence of mRNA synthesis Enzyme induction E. coli can synthesize ˜4300 polypeptides But enormous variation in abundance of specific polypeptides: Ribosomal protein: 10’000 copies/cell Regulatory protein: <10 copies/cell Housekeeping enzymes, constitutive Adaptive, inducible enzymes Lactose-metabolizing enzymes are inducible E. coli is initially unable to metabolize lactose, but starts to induce the corresponding enzymes: lactose permease, for uptake β-galactosidase, for splitting lactose Few copies -> >10% or proteins, 1000-fold Triggering substance: inducer, allolactose or IPTG The induction kinetics of β- galactosidase in E. coli The E. coli lac operon Structural genes Z, β-galactosidase Y, lactose permease A, thiogalactisodase transacetylase Control site Regulatory gene P, promoter I, inducer O, operator Bacteria can transmit genes via conjugation Conjugation allows for bacterial genetics Constitutive mutation: lac operon induced even without inducer -> mutation in I, distinct but closely linked to structural genes PaJaMo experiment, 1956, Arthur Pardee Francois Jacob, Jacques Monod Bacterial conjugation F– cell acquires an F factor from an F+ cell Bacterial conjugation: Transfer of genetic material Between a donor cell, F+, and recipient, F- Ability to conjugate/mate is encoded on a plasmid, F factor (fertility) F+ cell are covered with F pili Allows binding to F- cell surface Formation of cytoplasmic bridge and transfer of genetic information Converts F- to F+ Transfer of the bacterial chromosome from an Hfr cell to an F– cell and its subsequent recombination with the F– chromosome F factor can spontaneously integrate into the genome -> Hfr strain (high frequency of recombination) -> transmission of genomic information upon mating: in fixed order time dependent (90min) Merozygote, partially diploid Recombination and integration The PaJaMo experiment Mate Hfr I+Z+ to F- I-Z- in absence of inducer Monitor β-gal activity over time Induction after 1h, cessation upon 2h -> Z+ in I- cells leads to constitutive induction, cessation upon transfer of I gene -> I gene codes for diffusible repressor of Z, lac repressor F- is resistant to T6 and streptomycin Messenger RNA Second type of constitutive mutation Oc, operator constitutive, maps between I and Z genes In merozygote F’ Oc Z- / F O+ Z+, β-gal inducible But in Oc Z+ / F O+ Z- constitutive synth of β-gal O Can control Z only when on same chromosome !!! -> cis acting control I is trans acting factor Proteins are synthesized in two stages: 1. DNA is transcribed in mRNA 2. mRNA is translated into protein This model explains behavior of lac system Messenger RNA In the absence of inducer, I binds to O and represses synthesis of structural genes Z, Y, A On binding inducer, repressor dissociates from O, permitting transcription and subsequent translation Operator-Repressor-Inducer system represents a molecular switch Oc is constitutive because repressor cannot bind, Cis-acting element Coordination of all 3 protein by single polycistronic mRNA transcript, cistron mRNAs have their predicted properties Kinetic of enzyme induction -> mRNA has to be rapidly synthesized and degraded, short half-life rRNA turnover is slower, comprises 90% of cellular RNA The distribution, in a CsCl density gradient, of 32P-labeled RNA that had been synthesized by E. coli after T4 phage infection The hybridization of 32P-labeled RNA produced by T2-infected E. coli with 3H-labeled T2 DNA 2. RNA Polymerase RNA polymerase is responsible for the DNA-directed synthesis of RNA (1960), dNTP and DNA-dep. E. coli RNAP hplpenzyme, 459kD, αββ'ωσ subunit composition, sigma σ70 unit dissociates from core once RNA synthesis has been initiated RNAP functions: 1. Template binding 2. RNA chain initiation 3. Chain elongation 4. Chain termination Components of E. coli RNA Polymerase Holoenzyme Electron micrograph of E. coli RNA polymerase (RNAP) holoenzyme attached to various promoter sites on bacteriophage T7 DNA Template binding o RNA synthesis is initiated only at specific sites on the DNA template o RNAP binds to its initiation sites at sequence elements called promoter, these are recognized by sigma factor (K ≈10-14M) o Promoter, ca. 40bp element, located 5’ of structural gene, first base in RNA is +1, initiation site o If RNAP bound to promoter -20 to +20 are DNaseI protected o Consensus promoter sequence, hexamer centered at - 10 = Pribnow Box, TATAAT, plus additional element at - 35 sequence in between not important, but distance o +1 is either A or G The sense (nontemplate) strand sequences of selected E. coli promoters Rate of transcription varies 1000-fold, correlates with strength of promoter to bind RNAP Initiation requires formation of an open complex RNAP binding alters accessibility of bases towards methylating agents (dimethyl sulfate, DMS), DMS- footprinting -> holoenzyme binds to only one side/face of DNA and melts DNA Chain initiation +1 is purine, A > G Initiation reaction: pppA + pppN -> pppApN + Ppi Unlike DNA replication, RNA initiation does not need a primer Crab claw shape of Taq RNAP, pincers formed by β and β ’ with a cavern between the two pincers Prokaryotic initiation is inhibited by rifamycin B, Steroptomyces mediterranei, rifampicin commercial gram- positive antibiotic (also tubeculosis), does not block promoter bdg or elongation, but only initiation Structure of Taq RNAP core enzyme Chain elongation 5’ -> 3’ or 3’ -> 5’ growth ? Label with γ[32P]GTP, chase with cold GTP If 5’->3’, RNA is permanently labelled If 3’->5’, RNA is unlabelled Transcription supercoils DNA Elongation requires opening of dsDNA, bubble 2 models: RNAP swirls around DANN -> transcript would wrap DNA rotates -> DNA must be tethered Transcription occurs rapidly and accurately In vivo rate is 20-50nt/sec, entirely processive, no exo- nucleolytic correction like in DNA pol. One mistake per 10’000nt, tolerable because: 1. Genes are repeatedly transcribed 2. Genetic code is redundant -> high prob. of silent mut. 3. Aa substitutions in proteins are often tolerated 4. Large portion of transcript is non-coding (intron) Intercalating agents inhibit both RNA and DNA polymerase Actinomycin D intercalates into DNA and RNA, inhibits transcription and replication Chain Termination EM specific sites of termination, two common features in E. coli: 1. Series of 4-10 consecutive A-Ts, As on template 2. G + C-rich palindromic region upstream of A-Ts A hypothetical strong (efficient) E. coli terminator The stability of terminator G+C hairpin + weak base pairing Between RNA polyU and DNA template ensure termination Termination often requires the assistance of Rho factor 50% of termination sites lack cis-acting terminator sequences, but require protein factor, Rho in vivo transcripts often shorter than in vitro Rho is hexameric, 419Aa Helicase activity Recognition sequence on RNA transcript Eukaryotic RNA polymerase 3 distinct types of RNAP 1. RNAP I, in nucleoli, makes rRNA 2. RNAP II, in nucleoplasm, makes mRNA precursors 3. RNAP III, nucleoplasm, 5S rRNA, tRNA, small RNAs Up to 600kD, up to 12 subunits, 5 of these present in all 3 RNAP types RNAP II has extraordinary C-terminal domain, CTD 52 repeats of PTSPSYS, 50 Ser are phosphorylated Transcription is only initiated if CTD is unphosphorylated Elongation occurs only if CTD is phosphorylated Phosph. Converts initiation complex to elong. compl. RNA Polymerase Subunitsa X-Ray structure of yeast RNAP II that lacks its Rpb4 and Rpb7 subunits Resembles Taq RNAP crab claw like shape Cutaway schematic diagram of the transcribing RNAP II elongation complex On DNA binding, 50kD clamp swings out -> processivity Amatoxins specifically inhibit RNA polymerase II and III Poisonous mushroom, Amanitia phalloides Responsible for majority of fatal mushroom poisonings Toxin, bicyclic octapeptide, amatoxins, α-amanitin Tight 1:1 complex with RNAP, K 10-8M Act slowly, death after a few days -> turnover of RNA Mammalian RNAP I has a bipartite promoter Numerous rRNA genes have essentially identical sequence and promoter But unlike RNAP II and RNAP III, RNAP I promoters are Species specific !! Core promoter -31 to +6 and upstream element (-187 to -107) RNAP II promoters are complex and divers Euk RNAP II promoters are more complex than their prokaryotic homologues GC-box upstream of constitutive genes Selectiveley expressed genes often contain TATA box (-27 to -10), resembles -10 of prok. Genes Mutation sin TATA box cause heterogeneity in initiation CCAAT box, -70 tp -90, i.e. in globin genes
Details
-
File Typepdf
-
Upload Time-
-
Content LanguagesEnglish
-
Upload UserAnonymous/Not logged-in
-
File Pages120 Page
-
File Size-