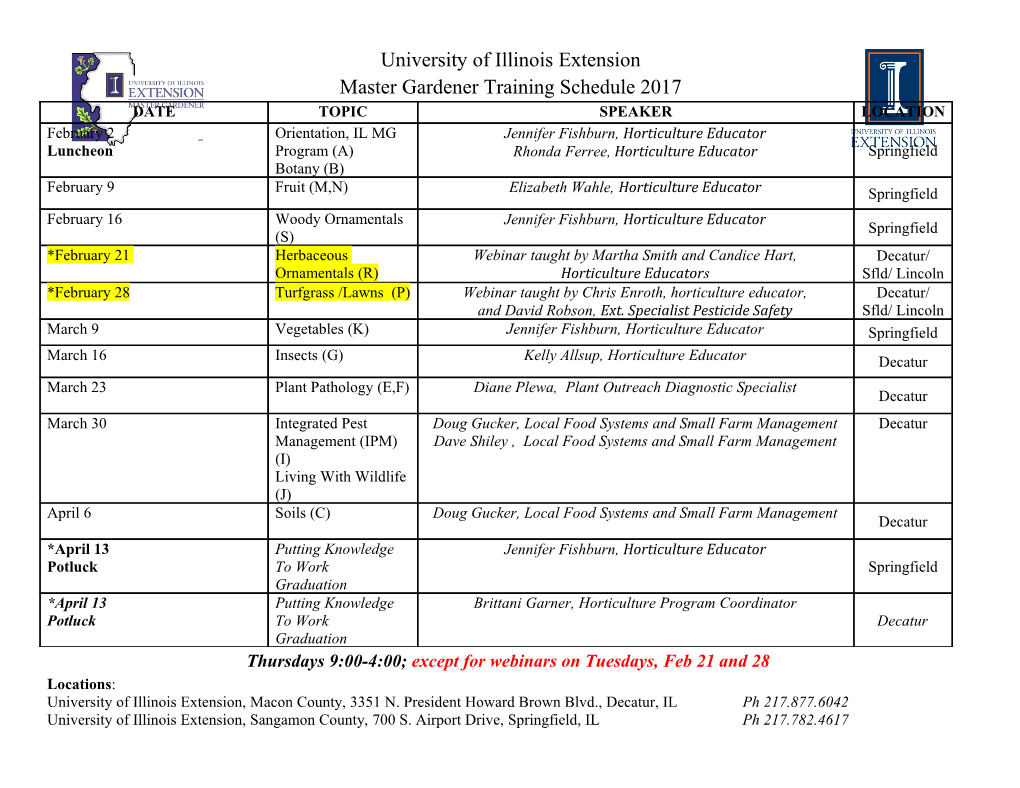
Evaluation of Material Attractiveness to Non-state Actors of Various Nuclear Materials in Thorium Fuel Cycles By Eva Lisowski Submitted to the Department of Nuclear Science and Engineering In Partial Fulfillment of the Requirements for the Degree of Bachelor of Science in Nuclear Science and Engineering At the Massachusetts Institute of Technology May 2020 © 2020 Massachusetts Institute of Technology. All rights reserved. Signature of Author: ………………………………………………………………………….. Department of Nuclear Science and Engineering May 12, 2020 Certified by: …………………………………………………………………………………... Benoit Forget Associate Department Head and Professor of Nuclear Science and Engineering Thesis Supervisor Certified by: …………………………………………………………………………………... Michael Short Associate Professor of Nuclear Science and Engineering Undergraduate Chair 1 2 Evaluation of Material Attractiveness to Non-state Actors of Various Nuclear Materials in Thorium Fuel Cycles By Eva Lisowski Submitted to the Department of Nuclear Science and Engineering on May 12, 2020, in partial fulfillment of the requirements for the degree of Bachelor of Science in Nuclear Science and Engineering Abstract Thorium-based fuel cycles for advanced nuclear reactors have been explored to utilize thorium resources in nations where uranium is scarce, increase fissile material utilization, and enhance proliferation resistance. As a stepping stone, thorium-based fuels have been paired with pressure tube heavy water reactors because of their high neutron economy and online refueling capability. However, thorium fuel cycles have raised proliferation concerns regarding the presence of U-233 following the irradiation of fuel bundles. The presence of Pa-233, which decays into pure U-233, and the creation of Pu-239 due to the neutron capture of U-238 in mixed lightly-enriched uranium (LEU)/Thorium fuels, are also causes for proliferation concern. Based on a method developed in a previous study, the material attractiveness to non-state actors of fissile materials present in a 40%LEU/60%Th fuel lattice concept was evaluated for six metrics: bare critical mass (BCM), heat content, net weight, acquisition time, dose rate, and processing time & complexity. The lattice, composed of 35 fuel pins and a central ZrO2 displacer rod, was modeled and depleted in the OpenMC reactor physics software, over a range of burnups up to 40 MWd/kg followed by two years of cooling. It was found that the material attractiveness of uranium isotopes in the irradiated fuel bundle was Very Low due to the high fraction of U-238 present in the fuel and the assumed lack of enrichment capabilities among non-state actors. However, for a state with basic enrichment capabilities, this fuel may be attractive. The attractiveness of plutonium isotopes was also found, as expected in a thorium-cycle, to be Very Low. However, the low BCM and heat content of this mixture reveals that it could be attractive to states that can easily acquire the material and do not need to rely on the theft of many fuel bundles to acquire an IAEA Category I quantity of material. Further investigation of the material attractiveness to states is required. Material attractiveness evaluations are important to informing future decisions regarding which fuel bundles to select when designing advanced reactor facilities and developing methods to safeguard them. Thesis Supervisor: Benoit Forget Associate Department Head and Professor of Nuclear Science and Engineering 3 Acknowledgments I would like to express special appreciation and gratitude to the people below who made the research and writing process successful and assisted me at every step towards my goal: Professor Forget, my thesis supervisor, for his vital support and assistance, for spending time to help me develop and better understand my calculation methodology, and providing me with both essential and bonus resources to allow me to successfully complete my project, and expand my knowledge and confidence in the field of reactor physics, and especially for having faith in my ability to meet project deadlines despite the extenuating circumstances under COVID-19. Professor Hiroshi Sagara, my lab supervisor during my fall 2019 semester study abroad at the Tokyo Institute of Technology, for welcoming me as a member of his lab, for introducing me to his research in nuclear non-proliferation and inspiring me to write a thesis based on his lab’s material attractiveness methodology, and for giving me the foundation for a successful research project. The MIT Computational Reactor Physics Group, led by Professor Forget, for supporting me financially by providing me with the computational resources needed to perform complex reactor physics calculations, and Google, which donates free computing credits for use of its Google Cloud Platform Compute Engine. Dr. Jiankai Yu, a postdoctoral fellow in the MIT Computational Reactor Physics Group, for his patience and sympathetic attitude at every point during my project, for training me in the use of OpenMC, helping me troubleshoot many software difficulties, and answering my many questions throughout the entire computation process. Professor Mike Short, my academic advisor, for his support throughout my academic career at MIT, for helping me through transitioning my initial research concept to a fully developed thesis idea, and for keeping me on track by advising me throughout the research process. Brandy Baker and Heather Barry, the NSE academic administrative staff members, for their tireless efforts in continuous support of NSE undergraduates, especially graduating seniors dealing with repercussions due to COVID-19, and for their reminders and motivation that encouraged me to meet project deadlines to the best of my ability despite the extenuating circumstances. My partner and best friend, Daniel, for his love and patience throughout the school year, and for his support and perseverance through long-distance separations, time zone differences, and clashing sleep schedules. Finally, I would like to thank everyone above for showing resilience, perseverance, and flexibility through remote communication and social isolation amidst the COVID-19 pandemic. 4 Table of Contents Abstract 3 Acknowledgements 4 Table of Contents 5 List of Figures 6 List of Tables 7 1. Introduction 8 1.1 Motivation 8 1.2 Objectives 9 2. Background 10 232 233 2.1 U/ U Ratio in Thorium Fuel Cycles 10 2.2 35-LEU/Th-ZrO2-rod Lattice Concept 14 2.3 Material Attractiveness 16 3. Methodology 19 3.1 Identification of Fuel Cycle & Materials 19 3.2 The OpenMC Monte Carlo Code 19 3.3 Model Thorium Fuel Bundle & Simulate Depletion 20 3.4 Material Attractiveness Evaluation of Direct-use Materials 23 4. Results and Discussion 26 4.1 Depletion Summary 26 4.2 Uranium NED: Material Attractiveness to Non-state Actors 28 4.3 Plutonium NED: Material Attractiveness to Non-state Actors 28 5. Conclusion 32 5.1 Future Options 32 Appendix A: Fuel Bundle Geometry and Depletion Code 34 Appendix B: Bare Critical Mass Calculation Code 40 References 42 5 List of Figures Figure 1: Products of multiple-neutron captures on Th-232. 12 Figure 2: Neutron capture cross section data for Th-231 production and Th-233 production. 13 Figure 3: 35-LEU/Th-ZrO2-rod Lattice Concept modeled in the reference study. 14 Figure 4: k-infinity vs. Burnup for the 35-LEU/Th-ZrO2-rod bundle concept and Maximum burnup for LEU/Th lattices. 15 Figure 5: Discrete phases in nuclear explosive device (NED) development. 16 Figure 6: Generic steps to make a uranium-based NED. 18 Figure 7: 35-LEU/Th-ZrO2-rod Lattice Concept modeled in OpenMC. 20 Figure 8: k-effective vs. Burnup for the 35-LEU/Th-ZrO2-rod bundle concept with 40% LEU modeled in OpenMC. 26 Figure 9: Number of atoms vs. Time [d] of uranium isotopes in the 35-LEU/Th-ZrO2-rod fuel bundle. 27 Figure 10: Number of atoms vs. Time [d] of plutonium isotopes in the 35-LEU/Th-ZrO2-rod fuel bundle. 27 6 List of Tables Table 1: Comparison of some nuclear characteristics of U-233, U-235, and Pu-239. 11 Table 2: Scales of categorization for the material attractiveness for non-state actors. 16 Table 3: Specifications for Thorium-based fuels in PT-HWRs. 21 Table 4: Definition of the Attractiveness Level Bins. 25 Table 5: Masses and isotopic fractions of plutonium isotopes in the 35-Th/LEU-ZrO2-rod Fuel Bundle after zero to 40 MWd/kg of depletion and two years of cooling. 29 Table 6: Estimation of the Heat Content of a bare critical sphere containing Pu-238, Pu-239, Pu- 240, Pu-241, and Pu-242. 29 Table 7: Overall material attractiveness evaluation of Plutonium isotope mixture. 31 7 1. Introduction As nations aim to develop clean energy sources and ensure energy security, new advanced nuclear reactor (ANR) concepts are becoming increasingly more attractive, along with innovative fuel cycles to complement them. In particular, thorium-based fuel cycles are becoming attractive in nations lacking an abundance of uranium resources, such as India, which contains over 800,000 tons, the largest reasonably assured resource of naturally-occurring thorium in the world [1], and has been working on designs for a thorium-based Advanced Heavy Water Reactor (AHWR) [2]. However, with new technologies come new weapons-proliferation vulnerabilities, and safeguard methods must evolve to address the possibility of theft and diversion of fissile material by non- state actors, such as terrorists. One method to evaluate the theft and diversion attractiveness level of nuclear materials was developed in a joint US-Japan study and published in 2013, and has been used primarily to examine materials in traditional Uranium-Plutonium fuel cycles [3]. This thesis aims to expand use of this method to begin to include thorium fuel cycles. 1.1 Motivation Prior to the development of this material attractiveness concept, studies explored how doping plutonium with different isotopic ratios could increase decay heat [4], one characteristic of fissile material that can make it difficult to steal, due to health risks.
Details
-
File Typepdf
-
Upload Time-
-
Content LanguagesEnglish
-
Upload UserAnonymous/Not logged-in
-
File Pages43 Page
-
File Size-