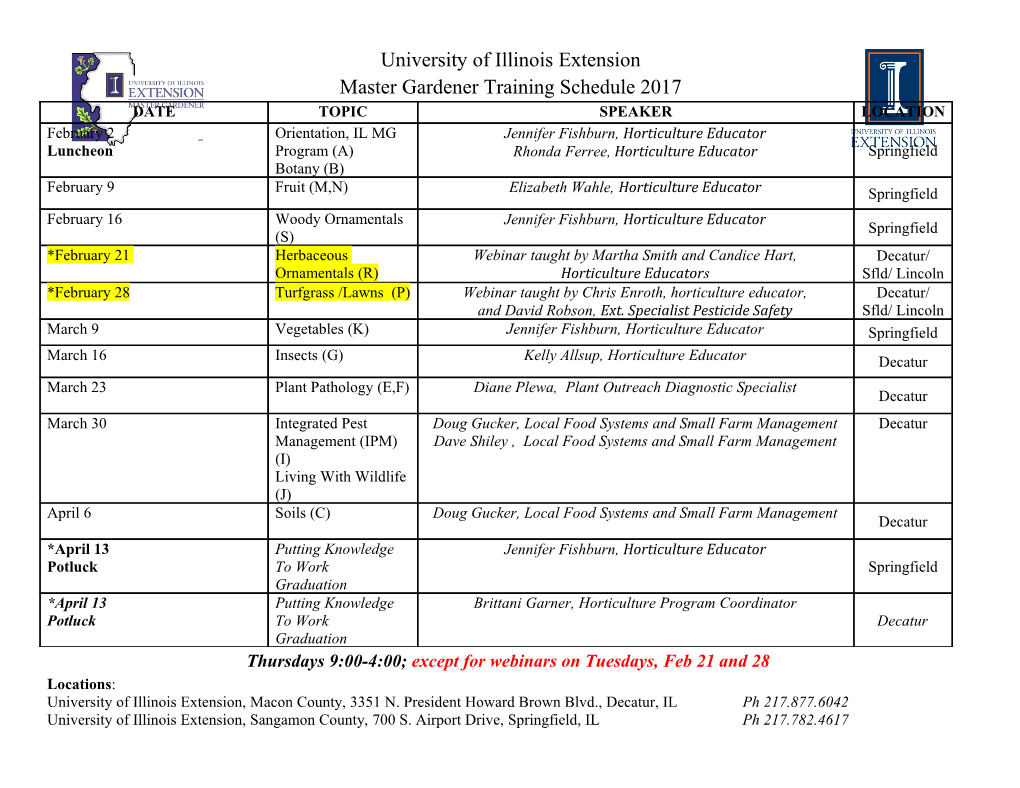
Vol. 66: 41–46, 2012 AQUATIC MICROBIAL ECOLOGY Published online March 14 doi: 10.3354/ame01553 Aquat Microb Ecol OPENPEN ACCESSCCESS Virus-driven nitrogen cycling enhances phytoplankton growth Emma J. Shelford1, Mathias Middelboe4, Eva F. Møller5, Curtis A. Suttle1,2,3,* 1Department of Earth and Ocean Sciences, 2Department of Botany, and 3Department of Immunology and Microbiology, University of British Columbia, Vancouver, British Columbia, V6T 1Z4, Canada 4Marine Biological Section, University of Copenhagen, 3000, Helsingør, Denmark 5National Environmental Research Institute, Aarhus University, 4000, Roskilde, Denmark ABSTRACT: Viruses have been implicated as major players in aquatic nutrient cycling, yet few data exist to quantify their significance. To determine the effect of viruses on ammonium regener- ation by bacteria, experiments were carried out in the oligotrophic Indian Ocean and productive False Creek, Vancouver, Canada. Bacteria were concentrated and then diluted with virus-free water to reduce virus abundance, or with virus-replete water to restore natural virus abundances. Virus-replete treatments showed increased ammonium concentrations compared to treatments with viruses removed (differences of 0.287 ± 0.14 and 1.44 ± 0.73 µmol l−1, mean ± SD, in the Indian Ocean and False Creek, respectively). Bacterial abundances were lower, while phyto- plankton abundances and chlorophyll a (chl a) concentrations were greater in the virus-replete treatments, consistent with the increased availability of ammonium in the presence of viruses. These data demonstrate that viral lysis leads to ammonium production, likely through the libera- tion of dissolved organic N that is remineralised by uninfected bacteria. In turn, the released ammonium fuels primary production. These results show that viruses play a critical role in the marine N cycle, and suggest that viral lysis likely supplies a significant portion of the global N requirements of phytoplankton. KEY WORDS: Ammonium · Virus · Bacteria · Remineralisation · Nitrogen Resale or republication not permitted without written consent of the publisher INTRODUCTION within the bacterial community. Viral loop models (e.g. Fuhrman 1992, Bratbak et al. 1994) incorporate Viruses are the most abundant biological entities in virus-induced cycling of carbon within the bacterial the ocean, of which the majority are thought to infect size fraction into the pelagic food web model, and bacteria (Suttle 2007). Every day, viruses lyse an esti- suggest that viral activity primarily acts as a sink of mated 20 to 40% of the bacterial production in the organic carbon by removing whole cells from grazing ocean (Suttle 1994), releasing bacterial cell contents and increasing bacterial respiration. This was veri- to the surrounding environment. This process has fied experimentally by Middelboe & Lyck (2002), in a been termed the ‘viral shunt’, to describe the viral- study in which viral activity stimulated carbon recy- mediated transfer of nutrients from particulate to dis- cling and respiration by uninfected bacteria, and solved material (Wilhelm & Suttle 1999, Suttle 2005). reduced the accumulation of microbial biomass. Fur- The lysis products are used by uninfected bacteria ther studies have confirmed efficient bacterial recy- (Middelboe et al. 1996, 2003); therefore, viruses play cling of carbon from bacterial (Middelboe et al. 2003) an important role in recycling carbon and nutrients or algal lysates (Haaber & Middelboe 2009). *Corresponding author. Email: [email protected] © Inter-Research 2012 · www.int-res.com 42 Aquat Microb Ecol 66: 41–46, 2012 There is also persuasive evidence that viruses are viruses are not simply parasites that disrupt food web important agents of N cycling in aquatic environ- flow (Azam & Worden 2004), but play a significant ments. Viral lysates are rich in free and combined role in nutrient recycling (Fuhrman 1999, Wilhelm & amino acids (Middelboe & Jørgensen 2006), and are Suttle 1999) and ultimately provide N that supports therefore a potentially important source of labile primary production. organic N. Moreover, bacteria assimilate dissolved organic nitrogen (DON) resulting from the lysis of infected microorganisms, especially when nutrient- MATERIALS AND METHODS limited (Gobler et al. 1997). During carbon-limited conditions, bacteria hydrolyse amino acids and other Description of sampling sites nitrogenous products to access the carbon, producing ammonium as a by-product (Goldman et al. 1987). Samples were collected from the surface waters of Assuming that the C:N ratio of viral lysates is similar False Creek (FC), Vancouver, Canada (49° 16’ N, to that of bacteria (i.e. between 4:1 and 5:1; Goldman 123° 7’ W) on 3 November 2008, and from 10 m depth et al. 1987) and that carbon growth efficiencies of in the Indian Ocean (IO) (19° 46’ S, 114° 52’ E) on 12 uninfected bacteria are in the range of 0.2 to 0.3 November 2006. The 2 study sites represented very (Middelboe et al. 1996), viral lysates contain N in different environments. The IO station is oceanic and excess of bacterial requirements. Consequently, bac- oligotrophic with a high degree of DOM recycling terial metabolism of viral lysates should produce (A. W. Visser et al. unpubl. data), and was character- inorganic N that is available to phytoplankton ized by very low concentrations of chlorophyll a (Haaber & Middelboe 2009). In addition to fuelling (chl a; 0.2 µg l−1), inorganic nutrients (nitrate, bacterial metabolism, viral lysis therefore may repre- 0.12 µmol l−1; phosphate, 0.08 µmol l−1; ammonium, sent a significant pathway for the regeneration of 0.16 µmol l−1), a sea surface temperature of 28°C, and ammonium, a major N source that fuels primary pro- a salinity of 35.0. In contrast, FC is a small productive duction in the ocean. inlet which is heavily influenced by neighbouring We hypothesise that viral lysis of bacteria releases English Bay, the Fraser River, and fresh water runoff cellular debris to the dissolved organic matter (DOM) from the City of Vancouver. Tidal mixing results in a pool, accessible to uninfected bacteria. The subse- vigorous exchange of water be tween the sampling quent turnover is potentially an important source of site at the mouth of FC and English Bay. Surface N for phytoplankton growth (e.g. Haaber & Middel- salinities in English Bay typically remain at ~25 to 26 boe 2009). Therefore, viral activity would not only be during November and December (Short & Suttle a carbon sink, but would also indirectly stimulate 2003), and temperatures range from ~7 to 10°C. primary productivity, and thus carbon production. These data are consistent with sea-surface tempera- The strongest evidence that viral lysis supports the tures for November 2008 of 10°C estimated from growth of phytoplankton was seen in several experi- satellite data (http://las.pfeg.noaa. gov/ oceanWatch/ ments conducted in the Gulf of Mexico and Medi- oceanwatch.php). In November, chl a was low terranean in which the growth rates and the propor- (0.47 µg l−1), and nutrient concentrations high (nitrate, tion of dividing Synechococcus cells were higher in 14.8 µmol l−1; phosphate, 1.43 µmol l−1; ammonium, treatments with viruses than in treatments in which 3.50 µmol l−1). viral concentration was either reduced by dilution with ultra-filtered seawater or was heat-inactivated (Weinbauer et al. 2011). Experimental design To determine whether ammonium regeneration associated with viral lysis influences phytoplankton The IO and FC experiments were designed to growth, an initial experiment was conducted in the examine the impact of viruses on ammonium produc- oligotrophic Indian Ocean and followed up with an tion and subsequent effects on phytoplankton experiment in False Creek, a small productive inlet growth. In both experiments, triplicate +V and −V by Vancouver, Canada. The experiments showed a treatments were set up with the objectives of keep- decrease of ammonium regeneration with removal of ing bacterial abundances at near in situ levels in both viruses, and a subsequent decrease in phytoplankton treatments, while reducing (−V) or maintaining (+V) growth. These data are significant because they not near in situ viral abundances. only quantify and verify the significance of viruses in In the FC experiment, water was filtered in series regenerating N, but also support the view that through 2.0 and 0.2 µm pore-size, 47 mm diameter Shelford et al.: Virus-driven nitrogen cycling 43 polycarbonate filters (AMD Manufacturing), with the cap tubes, kept in the dark, and frozen at −20°C. first filter removing larger phytoplankton and zoo- Chl a and ammonium were determined fluorometri- plankton, and the second concentrating bacteria cally following the respective protocols of Parsons et (Wilhelm et al. 2002). A transfer pipette was used to al. (1984) and Holmes et al. (1999). keep the bacteria in suspension above the 0.2 µm fil- ter. Viruses were removed using a Prep Scale-TFF Cartridge (Millipore) with a 30 kDa molecular weight Statistical methods cut-off. The −V treatment was prepared by adding 50 ml of bacterial concentrate to 450 ml of virus-free Differences of means were tested using 2-tailed water, and the +V treatment was prepared by adding Student’s t-tests. Equality of variance was tested 50 ml of bacterial concentrate to 450 ml of 0.2 µm fil- using an F-test. tered water. Incubations ran on a light:dark cycle of 14:10 h at in situ temperature, and subsamples were collected at time 0, 5.5, 10.25, 19, and 24.5 h. RESULTS The IO experiment was performed similarly; how- ever, water was filtered through a 20 µm pore-size Bacterial and viral abundances filter before bacteria were concentrated above a 0.2 µm pore-size Pellicon filter (Millipore), and Bacterial and viral abundances (mean ± SD) in the viruses were removed using a 47 mm diameter initial water samples at IO and FC were 8.61 ± 0.45 × 0.02 µm pore-size Anodisc filter (Whatman).
Details
-
File Typepdf
-
Upload Time-
-
Content LanguagesEnglish
-
Upload UserAnonymous/Not logged-in
-
File Pages6 Page
-
File Size-