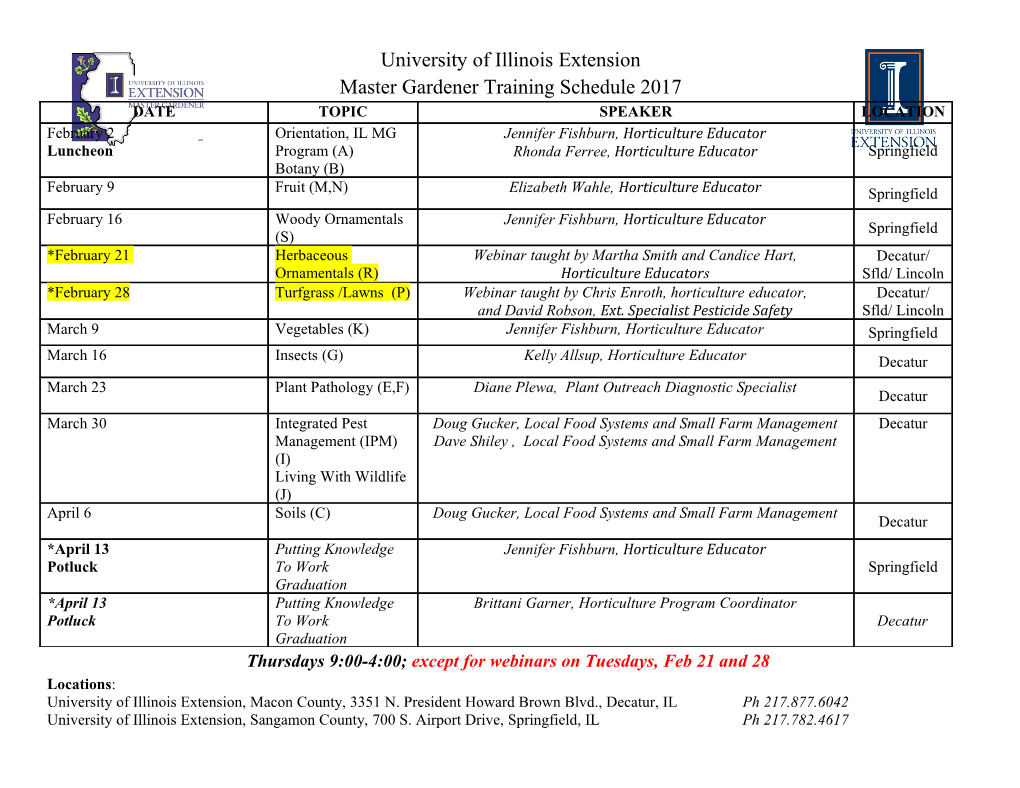
Regenerative cooling analysis of oxygen/methane rocket engines Luka Denies Delft University of Technology REGENERATIVE COOLING ANALYSIS OF OXYGEN/METHANE ROCKET ENGINES by Luka Denies in partial fulfillment of the requirements for the degree of Master of Science in Aerospace Engineering at the Delft University of Technology, to be defended publicly on Thursday December 10, 2015 at 15:30. Student number: 4056256 Supervisor: Ir. B. T. C. Zandbergen Thesis committee: Prof. dr. E. K. A. Gill, TU Delft Dr. ir. F.F.J. Schrijer, TU Delft Dr. R. Pecnik, TU Delft An electronic version of this thesis is available at http://repository.tudelft.nl/. REVISION HISTORY Revision Date Author(s) Description 1.0 2015-11-26 L. Denies Initial version provided to graduation committee 1.1 2016-01-11 L. Denies Updated captions of figures 5.26, 5.28 and 5.30-34 to clarify that the test article photo, experimental results and Fluent analysis are courtesy of CIRA iii ACKNOWLEDGEMENTS This thesis is the final milestone of my degree in Aerospace Engineering, marking the end of my time as a student in Delft. As such, I would like to thank those who helped me bring this graduation project to fruition, but especially also the people who have made my life the past six years an unforgettable experience. I owe gratitude to my supervisor, Barry Zandbergen, for his guidance, support and valuable feed- back. Through discussions with him, I was forced to think deeply about the narrative of this thesis, bringing much needed focus. I thank Ferry Schrijer and René Pecnik for their interest in my project, their expertise and their much appreciated advice. Marco Pizzarelli provided helpful comments by e-mail. I am deeply indebted to the people at CIRA in Italy for the access to their experimental data, but more importantly for their incredible hospitality. In particular, I want to thank Pasquale "il Festivo" Natale, Daniele Cardillo, Daniele Ricci, Marco Invigorito and Francesco Battista for their wonderful company, their help in rebuilding the test results, their expertise in CFD and for showing me their country. Grazie mille. To my many genootjes (in Dutch: afstudeerkamer-, beheers-, bestuurs-, commissie-, dispuuts-, huis-, ploeg-, senaats-, raads- en teamgenootjes) and other friends in Delft, I am grateful for making my time in Delft truly the time of my life. Because of them, my student time was a valuable experience in itself, rather than the means to an end. I want to thank my family for their unconditional support. Although I was rarely with them in Belgium, my mother, father and sister continued to support me in every possible way. Finally, my gratitude to Anouk, without whom it would all have been less meaningful. Thank you all. Luka Denies Delft, November 2015 v SUMMARY Methane is a promising propellant for future liquid rocket engines. In the cooling channels of a regen- eratively cooled engine, it would be close to the critical point. This results in drastic changes in the fluid properties, which makes cooling analysis a challenge. This thesis describes a two-pronged approach to tackle this problem. Simple and fast engineering tools allow for the development of insight in the design space using rapid iterations and parametric analyses. However, they are often rather inaccurate. In contrast, detailed multi-dimensional tools for numerical analysis are more accurate, but they require more computation time. Both approaches are developed for the analysis of regenerative cooling channels of oxygen/methane engines. Each ap- proach uses complex but accurate models for the thermodynamic and transport properties of methane. OMECA (short for One-dimensional Methane Engine Cooling Analysis) is a one-dimensional tool that was developed in Python from scratch. This tool divides a nozzle into stations and analyses the one- dimensional thermal equilibrium at each station. It makes extensive use of semi-empirical equations to calculate the heat transfer at both the hot gas side and the coolant side. The tool is compared to a coupled multi-physics analysis tool, showing that the accuracy of the wall temperature is rather poor, with discrepancies of up to 150 K. Both at the hot gas and coolant side, large deviations are present. However, if the input heat flux is correct, OMECA predicts the coolant pressure drop and temperature rise with a 10% accuracy. To obtain a higher accuracy at the coolant side, the open-source CFD package OpenFOAM is adapted for analysis of supercritical methane. Of particular note is the custom library that interpolates the fluid property tables at runtime. The selected solver is applicable to steady-state compressible flows. The software is then systematically validated using three validation cases. With experimental validation data obtained through cooperation with CIRA, an accuracy of 15 K for the wall temperature prediction is demonstrated. The pressure drop is predicted within 10%. Traditionally, the launcher industry uses copper alloys as wall material in regeneratively cooled com- bustion chambers. They offer a high allowable temperature and high thermal conductivity, but are also heavy and expensive. Recently, several companies have demonstrated aluminium combustion chambers. Aluminium alloys have weight and cost advantages, but have lower allowable temperature and thermal conductivity. The developed tools for cooling analysis are therefore employed to compare aluminium and copper for a generic 10 kN combustion chamber. It is discovered that a thermal barrier coating must be em- ployed to protect the hot gas side of an aluminium combustion chamber, otherwise regenerative cool- ing is not feasible. Even with such a coating, the pressure drop required to cool the coated aluminium chamber is three times higher than the pressure drop required for a copper chamber. A difference in pressure drop has effects on the vehicle level. A larger pressure drop in the cooling channel of a rocket engine necessitates a higher feed pressure. For a pressure fed engine, this means the tank must be stronger and heavier. It is found that even at modest fuel mass, the increase in tank mass is eight times as large as the decrease in engine mass offered by aluminium. This shows that using aluminium for the chamber wall is not advantageous with respect to copper for a pressure fed, regeneratively cooled, oxygen/methane rocket engine. vii CONTENTS 1 Introduction 1 1.1 Methane-fuelled rocket engines................................. 1 1.2 Rocket engine cooling....................................... 2 1.3 Supercritical fluids......................................... 3 1.4 Cooling channel analysis..................................... 5 1.5 Combustion chamber materials................................. 6 1.6 Research question......................................... 6 1.7 Research plan ........................................... 6 1.8 Thesis structure .......................................... 7 2 Fluid and solid property models9 2.1 Methane properties........................................ 9 2.2 Chamber wall properties..................................... 15 3 First order analysis 19 3.1 Computational setup....................................... 19 3.2 Verification and validation.................................... 28 3.3 Parametric analysis........................................ 34 3.4 Exploring cooling channel design................................ 43 3.5 Conclusion and recommendations............................... 47 4 Numerical method 49 4.1 Governing equations ....................................... 49 4.2 Pressure-velocity-density coupling............................... 52 4.3 Turbulence modelling....................................... 53 4.4 Roughness modelling....................................... 57 4.5 Discretisation ........................................... 59 4.6 Summary of numerical methods ................................ 62 5 Validation 63 5.1 Numerical analysis of heated methane............................. 63 5.2 Numerical conjugate heat transfer analysis.......................... 75 5.3 Experimental data......................................... 84 5.4 Effect of uncertainty on design ................................. 92 5.5 Conclusions of validation campaign.............................. 93 6 Results of CFD analysis 95 6.1 Nozzle channel analysis ..................................... 96 6.2 Nozzle channels with improved geometry........................... 103 6.3 Mass calculation and comparison ............................... 108 6.4 Summary of results ........................................ 109 7 Conclusions and recommendations 111 A OpenFOAM guidelines 113 A.1 Convergence............................................ 113 A.2 Solvers................................................ 113 A.3 Wall functions ........................................... 114 ix LISTOF FIGURES 1.1 Illustration of a rocket engine with regenerative cooling .................. 3 1.2 Indication of thermodynamic state of various propellants in cooling channels [10].. 3 1.3 Mechanisms of heat transfer enhancement, impairment, deterioration and recovery. Figure from [27] .......................................... 4 2.1 Methane phase diagram showing variation of density with pressure and temperature as well as critical point [43] ..................................... 10 2.2 Variation of methane specific heat cp with temperature at various pressures. Curves for data from NIST [50], markers for model implemented in Python.............. 11 2.3 Comparison between fluid property models programmed
Details
-
File Typepdf
-
Upload Time-
-
Content LanguagesEnglish
-
Upload UserAnonymous/Not logged-in
-
File Pages143 Page
-
File Size-