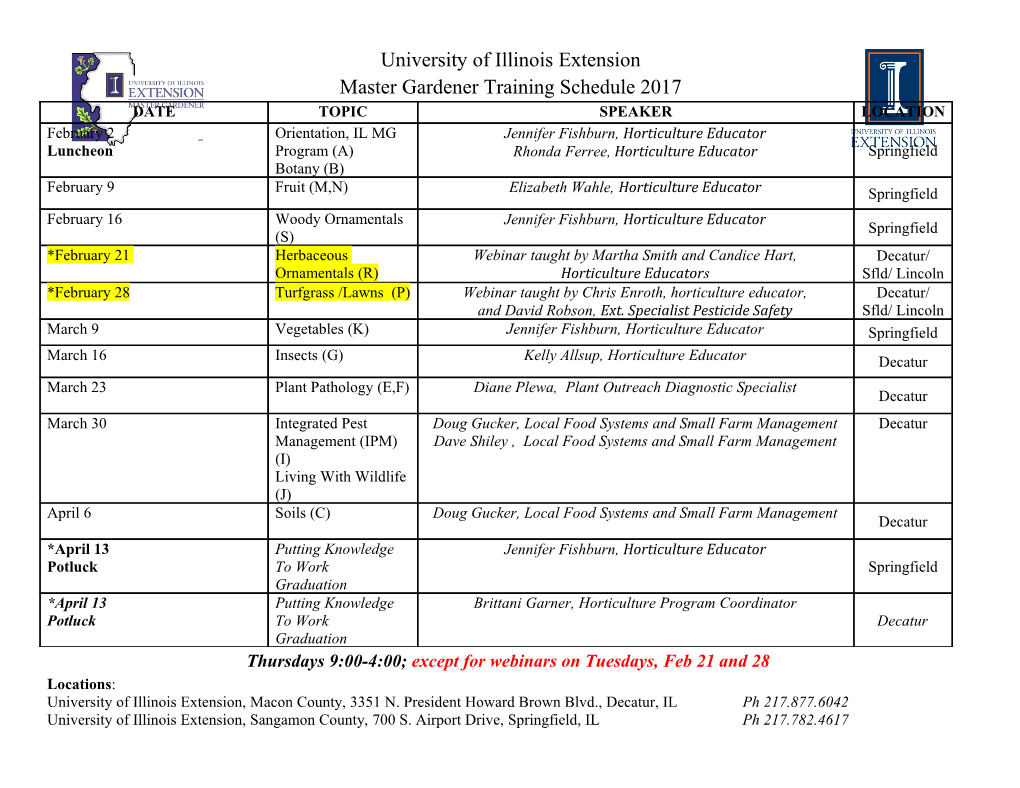
The Pennsylvania State University The Graduate School Intercollege Graduate Program in Plant Biology MAIZE DEFENSE RESPONSES AND STRATEGIES AGAINST ABOVEGROUND AND BELOWGROUND INSECTS A Dissertation in Plant Biology by Lina Castano-Duque © 2017 Lina Castano-Duque Submitted in Partial Fulfillment of the Requirements for the Degree of Doctor of Philosophy May 2017 The dissertation of Lina Castano-Duque was reviewed and approved* by the following: Dawn S. Luthe Professor of Plant Stress Biology Dissertation Adviser Kathleen M. Brown Professor of Plant Stress Biology Réka Albert Distinguished Professor of Physics and Biology Mary Barbercheck Professor of Entomology Committee Chair Teh-Hui Kao Distinguished Professor in Biochemistry and Molecular Biology Graduate Program Chair *Signatures are on file in the Graduate School. ii Abstract This dissertation had three main projects. In the first project we studied insect resistance traits against the root herbivore, western corn rootworm (WCR, Diabrotica virgifera) in the insect resistant maize genotype, Mp708 and its susceptible parent, Tx601 using biomechanical, root anatomy, molecular and biochemical analyses. These two maize genotypes differ in susceptibility to fall armyworm (FAW, Spodoptera frugiperda), WCR, and corn leaf aphid (CLA, Rhopalosiphum maidis). The data suggested that Mp708 is more resistant to WCR than Tx601 due to strong nodal roots, constant root growth under infestation, constitutive and induced expression of ribosomal protein 2 (rip2), terpene synthase 23 (tps23) and maize insect resistant cysteine protease-1 (mir1), as well high constitutive and inducible levels of MIR1-CP protein, jasmonic acid (JA) and caryophyllene. We concluded that Mp708 showed resistance and antibiosis against WCR and could be used as a model to explore the wide variety of mechanisms and traits involved in plant defense responses. In the second project we explored the use of innovative proteomic and network analyses to understand the global defenses deployed by maize plants infested with the root-feeder, WCR. Using the relatively new technique that employs 10-plex tandem mass spectrometry tags (TMT), we measured protein abundance changes in roots and leaves in response to these pest because metabolic changes in response to herbivory include a wide variety of mechanisms that enable the plant to survive. TMT data were analyzed by using a protein co-abundance and protein-protein interaction (PPI) networks. We detected 4878 proteins of which 863 had significant changes under WCR infestation. Proteins with higher abundance during WCR infestation were involved in the JA pathway and included lipoxygenase 5 (lox5), allene oxidase synthase 1 (aos1), and 12- oxophytodienoate reductase 2 (opr2). Other high abundance proteins during infestations were part iii of biosynthesis and signaling pathways for proteases of serine/cysteine type, abscisic acid, reactive oxygen species, and ethylene (ET). We validated changes in these pathways by analyzing the expression of key genes in each, total protease activity, cysteine and serine-type protease inhibition, JA and ET production. Our data showed promising differences between tissue-specific defense responses in leaves and roots of plants infested by WCR. Also, we suggested a dual insect and microbial plant stress response and postulated that roots infested with WCR are under constant microbial pressure due to the interactions in rhizosphere. In the third project we studied Mp708 tissue-specific responses to FAW by using the same proteomics and analysis techniques used for the second project. In addition we merged our proteomics network data with QTL known regions of resistance in Mp708 against FAW. We detected 4675 proteins of which 794 had significant changes under FAW infestation. Our analyses suggested an increase in the JA biosynthesis, REDOX changes in the glutathione and ascorbate pathways, and increase in ABA and ET biosynthesis and signaling. Infested leaf tissues showed high abundance and enrichment of proteinase inhibitors, cysteine proteases and peroxidases, these are well known plant defense proteins. Validations of total peroxidase activity showed an early activity spike in leaves of Mp708 and no change in the roots during FAW infestation , suggesting an early ROS signaling event that might not involve peroxide or an increase in quinone production mediated by peroxidases. Gene expression and phytohormone analyses showed that JA biosynthesis was activated in leaves and ET production increased only in roots, furthermore, JA and ET appears to control local MIR1-CP and RIP2 accumulation, and ET could be a key regulator of MIR1-CP systemic accumulation. These findings open up new routes in the hormonal control of local and systemic defense signaling that could be similar for other defense proteins and secondary compounds. Using the protein-correlation network with the iv known QTL regions, we found some proteins that might be part of the defense responses and tolerance against FAW that are involved in plant defenses, development and growth. Growth analyses determined that the insect susceptible maize genotype, Tx601, grew less in height and total root length during FAW infestation. We concluded that Mp708 defense mechanisms could involve lower trade-offs between plant growth and defense responses. We have established an analysis pipeline for proteomics data that includes network biology approaches that can be used with different types of “omics” data from a wide variety of organisms to detect tissue-specific defense responses. v TABLE OF CONTENTS List of Tables……………………………………………………………………………………viii List of Figures…………………………………………………………………………………. ...ix List of multimedia items………………………………………………………………………….xi Acknowledgements………………………………………………………………………………xii CHAPTER I. Introduction.........…………………………………………………………………. 1 CHAPTER II. A maize inbred exhibits innate resistance to the western corn rootworm, Diabrotica virgifera……………………………………………………….………………………5 Introduction…………………………………………………….………………………………….5 Materials and methods……………………………………………………….……………………9 Results……………………………………………………….…………………………………...15 Discussion……………………………………………………….……………………………….20 CHAPTER III. Plant Bio-Wars: Protein Networks Reveal Tissue-Specific Defense Strategies in Maize in Response to a Root Herbivore…………………………………………………………37 Introduction…………………………………………………….………………………………...37 Materials and methods……………………………………………………….…………………..42 Results and Discussion………………………………………………….……………………….54 Conclusions……………………………………………………….……………………………71 CHAPTER IV. Protein Networks Reveal Tissue Specific Defense Strategies in Maize in response to an aboveground herbivore…………………………………………………………………….96 Introduction…………………………………………………….………………………………...96 vi Materials and methods……………………………………………………….…………………..99 Results and Discussion………………………………………………….……………………...111 Conclusions……………………………………………………….…………………………….129 CHAPTER V. Conclusions…………………………………….……………………………….163 Bibliography…………………………………….………………………………………………………167 vii Lists of Tables Table 3.1. Proteins of interest from correlation network hotspot………………………………83 Table S3.1. Proteins identified from WCR TMT……...……………………………………….95 Table S3.2. Proteins present in the hierarchical clustering…………………………………….95 Table S3.3. Proteins in the abundance correlation network……………………………………95 Table S3.4. Proteins in the protein-protein interaction network……………………………….95 Table 4.1. Proteins that are part of the gene ontology (GO) term: “enzyme activity” ……….136 Table 4.2. Proteins that are part of the gene ontology (GO) term: “response to stimuli” ……137 Table 4.3. Proteins that belong to the subnetwork created from the protein correlation network, that fall within a known QTL for FAW……...………………………………………………...144 Table 4.4. Proteins of interest from the Hub from the protein abundance correlation network.147 Table S4.1. Identified proteins present in FAW TMT…………………………………………161 Table S4.2. Proteins and gene ontology annotation from the hierarchical clustering analysis...161 Table S4.3. Proteins in the abundance correlation network……………………………………161 Table S4.4. Proteins in the protein-protein interaction network……………………………….162 viii List of Figures Figure 2.1. Root length in Mp708 and Tx601 infestated with WCR……………………………26 Figure 2.2. Laser ablation tomography (LAT) cross-sections…………………………………..28 Figure 2.3. Time course analysis of aos and opr7………………………………………………30 Figure 2.4. Analysis of constitutive jasmonic acid (JA)..………………………………………31 Figure 2.5. Time course of rip2 and mpi...........................………………………………………32 Figure 2.6. Time course analysis of fpps3 and tps23.....………………………………………...33 Figure 2.7. Time course of mir1 transcript and MIR1-CP protein……………………………...34 Figure S2.1. Percent survival of WCR fed on Mp708 and Tx601 maize lines………………….35 Figure S2.2. Maximum cutting strength in lateral roots………………………………………...36 Figure 3.1. Hierarchical clustering of protein abundance ratios of Mp708…………………….74 Figure 3.2. Gene ontology (GO) hierarchical maps……………………………………………76 Figure 3.3. Gene ontology annotation that belong to Group 5………………………………..78 Figure 3.4. Betweenness centrality distribution of the correlation network.…………………..80 Figure 3.5. Hotspots from protein correlation and PPI networks……………………………...81 Figure 3.6. Node degree distribution of the PPI network………………………………………84 Figure 3.7. Gene ontology of PPI network.…………………………………………………….85 Figure 3.8. Analysis of salicylic acid (SA) and ethylene (ET) levels…………………………..87 Figure 3.9. Analysis of protease and inhibitors
Details
-
File Typepdf
-
Upload Time-
-
Content LanguagesEnglish
-
Upload UserAnonymous/Not logged-in
-
File Pages209 Page
-
File Size-