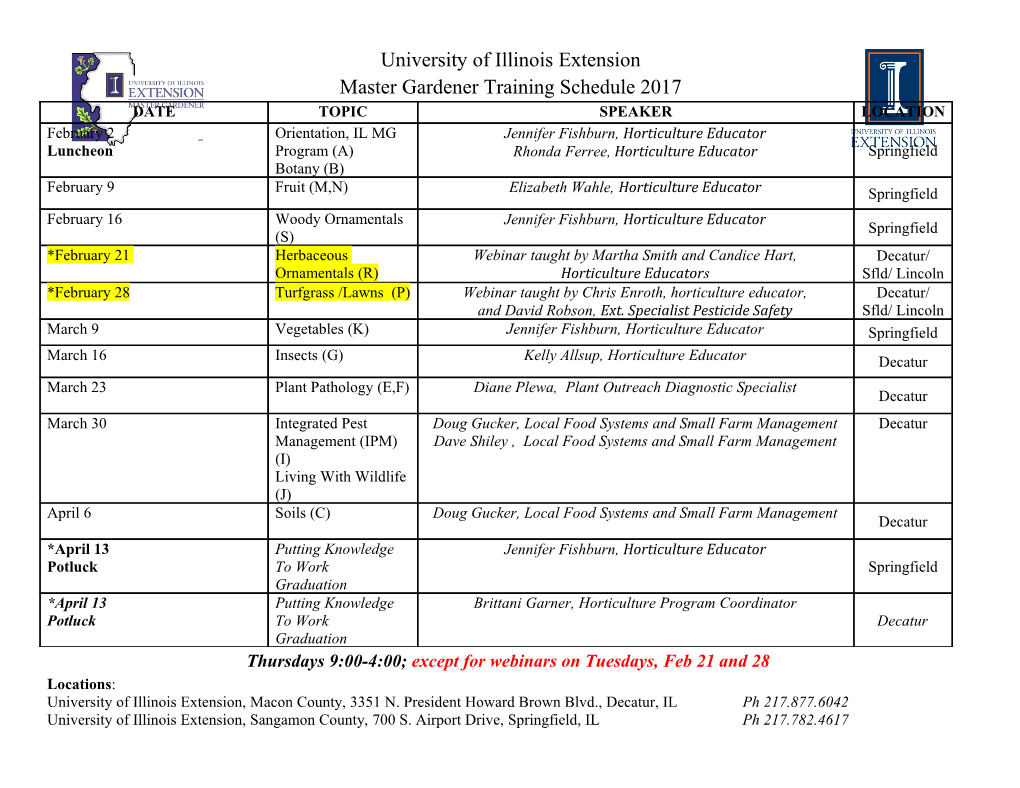
6.20 Six-membered Rings with 1,2,4-Oxygen or Sulfur Atoms CHARLES W. JEFFORD University of Geneva, Switzerland 6.20.1 INTRODUCTION 861 6.20.2 THEORETICAL METHODS 862 6.20.2.1 Comparison of Calculated and Experimental Parameters 862 6.20.2.2 Calculated Molecular Parameters as an Index of Antimalarial Activity 864 6.20.3 EXPERIMENTAL STRUCTURAL METHODS 865 6.20.3.1 Crystal Structures 865 6.20.3.2 NMR Spectroscopy 866 6.20.3.3 Raman Spectroscopy 867 6.20.4 THERMODYNAMIC ASPECTS 867 6.20.4.1 Thermal Stability 867 6.20.5 REACTIVITY 868 6.20.5.1 Thermolysis Reactions 868 6.20.5.2 Reactions with Base 871 6.20.5.3 Deoxygenation 872 6.20.5.4 Re due tion and Hydrogena tion 873 6.20.5.5 Reaction with Acid 873 6.20.5.6 Reaction with Ferrous Ion, Ferric Ion, Heme, and Hemin 877 6.20.6 SYNTHESIS 881 6.20.6.1 Addition of Singlet Oxygen to a-Pyrans and cc-Pyrones 881 6.20.6.2 Addition of Triplet Oxygen to 1,4-Diradicals 881 6.20.6.3 Addition of Singlet Oxygen to Monoalkenes in the Presence of Aldehydes 883 6.20.6.4 Addition of 1,2-Dioxetanes to Carbonyl Partners 885 6.20.6.5 Addition of Hydroperoxides to Carbonyl Partners 888 6.20.6.6 Addition of Endoperoxides to Carbonyl Partners 892 6.20.6.7 Cycloaddition and Dimerization 894 6.20.6.8 Miscellaneous Methods 895 6.20.7 IMPORTANT COMPOUNDS AND APPLICATIONS 898 6.20.1 INTRODUCTION There are eight possible ways of substituting oxygen and sulfur atoms at the 1-, 2-, and 4-positions within a cyclohexane ring (l)-(8). However, nearly all compounds reported are derivatives of 1,2,4- trioxane (1). About two dozen 1,2,4-trithianes are known, which are mostly simple alkylated derivatives of (2). Less than 30 compounds have been described for the remaining arrangements, 861 862 Six-membered Rings with 1,2,4-Oxygen or Sulfur Atoms namely for derivatives of 1,4,2- and 1,5,2-dioxathiane (3) and (4), 1,3,4-, 1,2,4- and 1,2,5-oxadithiane (6), (7), and (8). Derivatives of 1,2,4-dioxathiane (5) are unknown. S O > I ° O k .S L .0 k .0 (1) (2) (3) (4) 0' > S ^ O^i S o (5) (6) (7) (8) In comparison with their lower homologues, the 1,2,4-trioxolanes or secondary ozonides, tri- oxanes have received considerably less attention. No mention was made of them in the literature before 1957 <57JOC1682>. Thereafter, in the period 1957-1982, citations were sporadic. However, with the discovery in 1979 that a naturally occurring tetracyclic 1,2,4-trioxane, qinghaosu, or artemisinin (9), possesses potent antimalarial activity <79MI 620-01) the development of synthetic methods for preparing derivatives of (1) and (9) has grown dramatically. Contrary to expectation, most trioxanes are thermally stable, thereby permitting studies on their chemical and physical properties to be undertaken. The synthesis and chemistry of (9) <91H(32)1593, 94ACR2H) and of structurally simpler trioxanes <88HOU(E 13)720, 95COS225) have been reviewed. Accounts of (9) and its importance as an antimalarial have appeared <(85SCI1O49, 87MI 620-01, 92CSR85). The mechanistic principles underlying syntheses of bicyclic 1,2,4-trioxanes have also been reviewed (B-86MI620-01, B- 91MI620-01,93CSR59>. Less has been reported on (2). The first instance of an accidental synthesis of a 3,3-spirocyclic 1,2,4-trithiane was noted in 1970 <70JOC2102>. Since then, 3-alkyl derivatives of (2) have been identified as some of the exiguous ingredients responsible for the flavor of cooked meat <93MI 620- 01). Artificial foodstuff flavors, for example, those concocted by heating cysteine with sugar, contain the same derivatives <8lMl 620-01). The role of 1,2,4-trithianes and other heterocycles as flavor principles in processed foodstuffs has been reviewed <78H(l 1)663, B-78MI620-01). Allusions to mixed heterocycles (3)-(8) have been surprisingly few since the discovery that p- naphtha-1-thioquinone exists as the dimer, the first example of a 1,3,4-oxadithiane <3OJCS174O). Although theoretical considerations and physical measurements came after the molecules were prepared, the material in this chapter is presented in the usual format. However, there are some inevitable exceptions which spring from the fact that practically all the compounds discussed are fully saturated, nonnitrogen containing heterocycles. In other words, there is none of the traditional reactivity pertaining to unsaturated compounds, or fused benzene rings. Benzo derivatives are encountered only because of the particular method of preparation. Moreover, there are no sub- stituents attached to ring heteroatoms and no attendant reactivity. Synthesis is necessarily confined to the different ways in which the six-membered ring may be assembled. In other words, the chemistry of these heterocycles is reminiscent of that of alicyclic compounds, but evidently dominated by the peroxidic functionality. Furthermore, the present chapter did not feature in the first edition of Comprehensive Heterocyclic Chemistry (CHEC-I) and is therefore new in substance and, to a certain extent, new in style. 6.20.2 THEORETICAL METHODS 6.20.2.1 Comparison of Calculated and Experimental Parameters Theoretical methods have been used to compute geometries, heats of formation, conformational energies, and molecular electrostatic potentials (MEPs) of certain derivatives of (1) and (9). Deriva- Six-membered Rings with 1,2,4-Oxygen or Sulfur Atoms 863 tives of (2)-(8) have not received such attention. Calculation is often performed in the context of testing the accuracy of a particular method or in association with a physical measurement. l,2,4-Trioxan-5-ones provide an illustration. Despite appearances, such molecules are thermally stable. The cis- and trans-3,6 disubstituted (10) and (11) and the doubly spirocyclic (12) derivatives are crystalline substances, the structures of which have been determined by x-ray <91AX(C)388>. Their experimental geometries have been used as yardsticks for evaluating the accuracy of molecular mechanics (MM2) and semiempirical (AMI, PM3) methods <95JST(337)3l>. X-ray analysis shows that the trioxane ring exists as an envelope in (10) and as half-chair conformations in (11) and (12) <91AX(C)388>. O o (10) (11) All three computational methods give global minima corresponding to half-chair conformations for all three trioxanones. Calculated and measured bond lengths, bond angles and dihedral angles of the trioxanone portion have been compared. As expected, the greatest discrepancies are observed for the O—O bond. PM3 overestimates and AMI underestimates its length, whereas MM2 gives a result little different from the actual value. All three methods predict the other C—O and C—C bond lengths with the same degree of high accuracy. Calculation performs best for (10) and (11). Although the conformation of (10) is not that predicted by MM2, calculated bond angles for (10)- (12) could be usefully compared. The angles O(l)-O(2)-C(3) and O(4)-C(5)-C(6) are too wide when calculated by AMI and PM3. All three methods poorly predict the O(4)-C(5)-O(7) angle. Excepting (10), calculated and experimental values for the dihedral angles in (11) and (12) are in good agreement. Overall, MM2 is found to be the most accurate calculation method, with PM3 being slightly more accurate than AM 1. Calculations have also been carried out on the cyclopentene-fused 1,2,4-trioxane (13) <95HCA647>. The potential energy surface of (13) has been explored. Full geometry optimization was performed with the CHEM-X and MOPAC programs by using1 the PM3 method. The global minimum is computed to have a heat of formation of 0.9 kcal mol" and a flattened, twisted chair conformation (Figure 1, TC). Two local minima are found nearby, one in which the trioxane ring exists as a twist- boat (TB) and another1 as a twist-chair conformer (TC). Passage between TC and TB is easy, requiring 9.8 kcal mol" to reach the transition structur1 e (ts-1). Similarly, transit from TB through ts-2 to TC' is even easier, needing only 2.3 kcal mol" . Ph o-o -Ph (13) The aforementioned theoretical conformational mobility of the ds-fused bicyclic entity is con- firmed by line shape analysis of the NMR spectru# m of (13) in 1the temperatur# e rang1e 223-271 3 K. Th#e observed activatio1 n parameters, AH 3 = 15.2 kcal mol" , AS = 6.6 cal mol" K" and AG = 13.4 kcal mol" , are consonant with22 the equilibrium between conformers resembling TC and273 TB or TC'. The crystal structure of (13) confirms that the ground state is similar to TC. The 1,2,4-trioxane ring adopts a slightly flattened chair conformation bearing the angular phenyl substituent in the axial position (95HCA647). Semi-empirical and ab initio calculations have been performed on 5-hydroxymethyl-3,5,6,6-tetra- methyl-1,2,4-trioxane (14) <9llJQ23l>. A comparison of the AMI, PM3,3-21G, and 6-31G optimized geometries, all of which are chair conformations, are in excellent agreement with the x-ray structure. Similar comparisons have been made for artemisinin (9) <94UQll). Agreement between the ab initio 3-21G and 6-31G* calculations is excellent; both give 0(1)—0(2) bond lengths close to the value determined by x-ray. The trioxane ring assumes a twist-boat conformation and the Ol—02 bond length calculated by 3-21G (1.462 A) lies close to the experimental value (1.478 A). This result shows 864 Six-membered Rings with 1,2,4-Oxygen or Sulfur Atoms t.s.-2 o TC O Figure 1 Perspective views of the PM3-optimized geometries of conformers TC, TB, and TC and of the transition-state structures ts-1 and ts-2 of (13).
Details
-
File Typepdf
-
Upload Time-
-
Content LanguagesEnglish
-
Upload UserAnonymous/Not logged-in
-
File Pages39 Page
-
File Size-