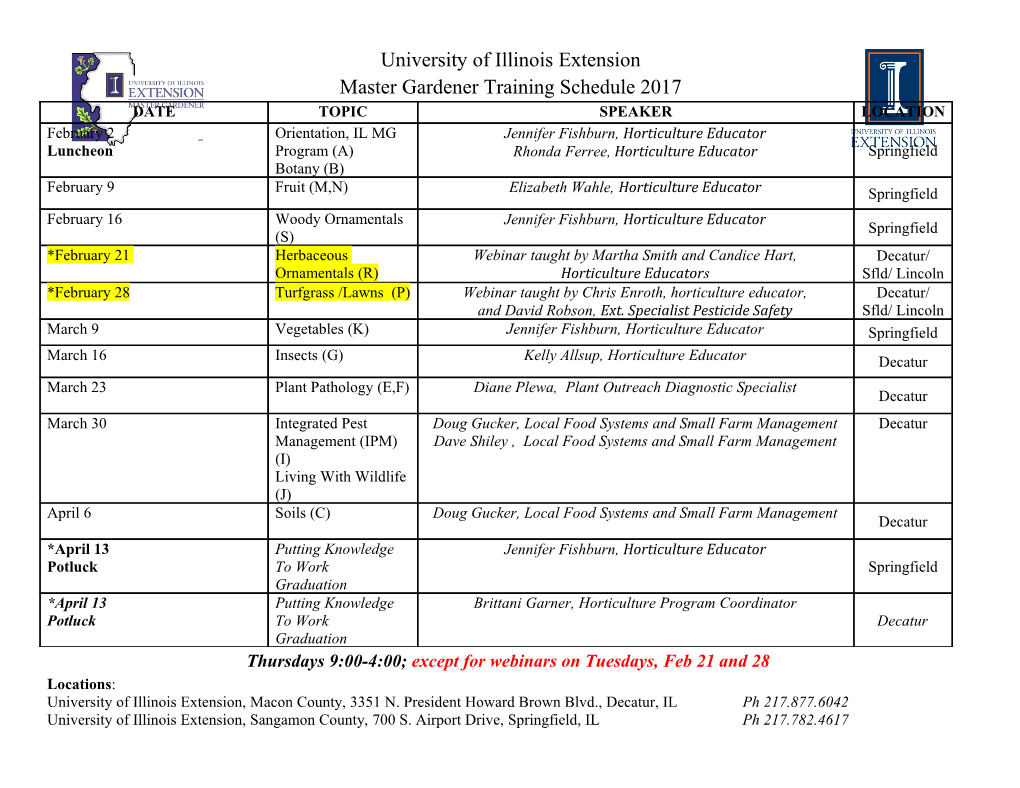
Old Dominion University ODU Digital Commons Physics Theses & Dissertations Physics Spring 2004 Neutron Star Electromagnetic Field Structure Hugh O. Thurman III Old Dominion University Follow this and additional works at: https://digitalcommons.odu.edu/physics_etds Part of the Stars, Interstellar Medium and the Galaxy Commons Recommended Citation Thurman, Hugh O.. "Neutron Star Electromagnetic Field Structure" (2004). Doctor of Philosophy (PhD), thesis, Physics, Old Dominion University, DOI: 10.25777/m1nr-v189 https://digitalcommons.odu.edu/physics_etds/70 This Thesis is brought to you for free and open access by the Physics at ODU Digital Commons. It has been accepted for inclusion in Physics Theses & Dissertations by an authorized administrator of ODU Digital Commons. For more information, please contact [email protected]. NEUTRON STAR ELECTROMAGNETIC FIELD STRUCTURE by Hugh O. Thurman III B.S. Physics May 1996 Old Dominion University M.S. Physics May 2003 Old Dominion University A Dissertation Submitted to the Faculty of Old Dominion University in Partial Fulfillment of the Requirement for the Degree of DOCTOR OF PHILOSOPHY PHYSICS OLD DOMINION UNIVERSITY May 2004 Appco/ed by: Gar^E. Copel^d (Director) Charles I. Sukenik G i l ^ R. Ho^ Rocco Schiavilla Jonh Adam Reproduced with permission of the copyright owner. Further reproduction prohibited without permission. ABSTRACT NEUTRON STAR ELECTROMAGNETIC FIELD STRUCTURE Hugh O. Thurman III Old Dominion University, 2004 Director: Dr. Gary E. Copeland This dissertation investigates the neutron star magnetic field from generation to radiation production. We have investigated the spontaneous magnetization process to explain the magnetic field generation. This magnetization is then applied to de­ termine the electromagnetic field structure of the neutron star. As an application of these two calculations, we briefly investigate several radiation mechanisms that are closely related to stellar magnetic fields. Neutron star magnetic field generation is studied through the spontaneous mag­ netization process. This process was studied in the non-relativistic, ultra-relativistic, and rigorous relativistic dispersion regimes for the neutrons. Both analytical and numerical approaches show that a phase transition is present for a density near and a temperature near 10®A'. This density is consistent with most neu­ tron star models. Using the magnetized interior, the neutron star electromagnetic field is derived from the vector potential. The derived magnetic field is more complicated than just a magnetic dipole which is the most common approximation to the magnetic field. The electromagnetic field structure is derived under the Goldreich-Julian approach. Finally this electromagnetic field is applied to three radiation mechanisms in at­ tempt to understand the high-frequency radiation observed from neutron stars. The processes studied are curvature radiation, pair production, and synchrotron radia­ tion. The curvature radiation is most greatly affected by the electromagnetic field because the radius of curvature is reduced by a factor 10 when just the quadrapole term is included. This directly affects the number of photons energetic enough to undergo pair production. These electron-positron pairs are also more energetic and the synchrotron radiation spectrum is affected by not only the injection angle but the magnetic field curvature as well. Reproduced with permission of the copyright owner. Further reproduction prohibited without permission. ©Copyright, 2004, by Hugh O. Thurman III, All Rights Reserved 111 Reproduced with permission of the copyright owner. Further reproduction prohibited without permission. ACKNOWLEDGMENTS I would like to thank several people for supporting me throughout my college career. First, 1 must thank my mother and father for helping me achieve my dream. Second, I must thank Neil and Tod for always making things fun and interesting in undergraduate school. Third, 1 must thank Walter, George, and Pasad. You guys made graduate school tolerable. Fourth, I must thank several professors: Dr. Copeland, Dr. Havey, Dr. Sukenik, Dr. Adam and Mr. Hanna. IV Reproduced with permission of the copyright owner. Further reproduction prohibited without permission. DEDICATION This dissertation is dedicated to the people who I truly respect for many different reasons. They include Neil, George, Pasad, Liz, Robin, Stan, and Mike. You each have taught me something and 1 am deeply grateful to each and every one of you for the brief moment in time we were able to share. I hope that every person could be as lucky as 1 have been to meet and know such great people as yourselves. Reproduced with permission of the copyright owner. Further reproduction prohibited without permission. PREFACE In 1933, Baade and Zwicky [1] introduced at the December American Physical Society meeting a new term in astrophysics, the supernova. This term referred to the cataclysmic event associated with the end of a massive star’s evolution. They also predicted the creation of a new stellar object from such an event, a neutron star. They proposed that this new stable stellar object would be composed completely of neutrons. This announcement followed Chadwick’s discovery of the neutron one year earlier in 1932. Baade and Zwicky were not the only ones to discuss a stellar object being composed of stable neutron matter. In 1932, Landau published a paper discussing the possibilities of stable neutron cores in massive stars [2]. He, however, did not predict a separate stable object. This announcement marked the beginning of a new area of physics research that is still active today. Neutron star research continued in 1939 with Oppenheimer and Volkoff’s equa­ tion of state for a neutron star [3]. Their equation of state incorporated general relativity and is considered still today one of the best models because of its ability to predict physical properties. As promising as their equation of state was, it also shed light on how difficult detection of these objects would be. Their predicted size was roughly 10 kilometers in radius with a surface temperature roughly a million degrees Kelvin. So even though the neutron star is extremely hot, it would be difficult to detect due to its low luminosity given its surface area and the fact that its maximum intensity wavelength output would be in the x-ray regime. So it appeared that these objects would be very difficult to detect. As the search for neutron stars continued, the advancement of x-ray astronomy grew as well. In 1962, Giaconni discovered the first x-ray source [4]. This discovery sparked a new interest in neutron stars because x-ray sources could now be detected. This excitement later faded when the x-ray source was determined to be a quasar. Quasars are considered quasi-stellar objects and maybe some of the most distant objects in our universe. They are useful in determining distances and the age of the universe. They, however, did not help in the discovery of neutron stars. Again it seemed these objects would never be detected. As the search for neutron stars continued, the next discovery was confusing at first, but, eventually a new theory was able to describe the observations. In 1967, VI Reproduced with permission of the copyright owner. Further reproduction prohibited without permission. Anthony Hewish and his graduate student, Jocelyn Bell, started their work on mea­ suring the cosmic background radiation using the Cambridge radio telescope [5]. As they collected data. Bell noticed a strong, regular pulse was continually present in one section of the sky. After eliminating all terrestrial sources, Hewish determined that the source must be extra-terrestrial. Thinking that the radio signals were from another intelligent life source, he called them LGM’s (Little Green Men). Hewish would later discover that the objects were stellar in nature and therefore could not be signals from an extra-terrestrial civilization. Due to the objects regular pulsing nature, these objects were considered to be pulsating stars. The name was shortened to pulsars. In 1968, Gold proposed that pulsars could be described as rotating magnetic neutron stars [6]. By looking at the radio spectra, the rotation rates were determined. Using the Oppenheimer-Volkoff predicted radius and mass, Gold determined that only neutron stars would able to withstand these rotational rates. He determined that white dwarf stars would disintegrate if they were rotating at these high rotational periods. With the discovery of these new objects called pulsars, neutron stars were finally detected using radio astronomy. This propelled a new era of neutron star research which is still strong even after 35 years of work. Many questions are left to be answered about these strange stellar objects. This area of physics has been so pro­ ductive that it has produced two Nobel Prizes in Physics. In 1974, Anthony Hewish won the Nobel Prize for his discovery of pulsars. In 1986, J.H. Taylor and J.M. Weisberg won the Nobel Prize for their work with binary pulsar systems and the verification of Einstein’s theory of general relativity [7]. Taylor and Weisberg were able to deduce the mass of each pulsar using Einstein’s theory and the regular timing of the pulsar pulses. The masses they calculated were near the Ghandrasekar limit of 1.4 solar masses [8]. V I1 Reproduced with permission of the copyright owner. Further reproduction prohibited without permission. Vlll TABLE OF CONTENTS Page List of Tables .................................................................................................................
Details
-
File Typepdf
-
Upload Time-
-
Content LanguagesEnglish
-
Upload UserAnonymous/Not logged-in
-
File Pages208 Page
-
File Size-