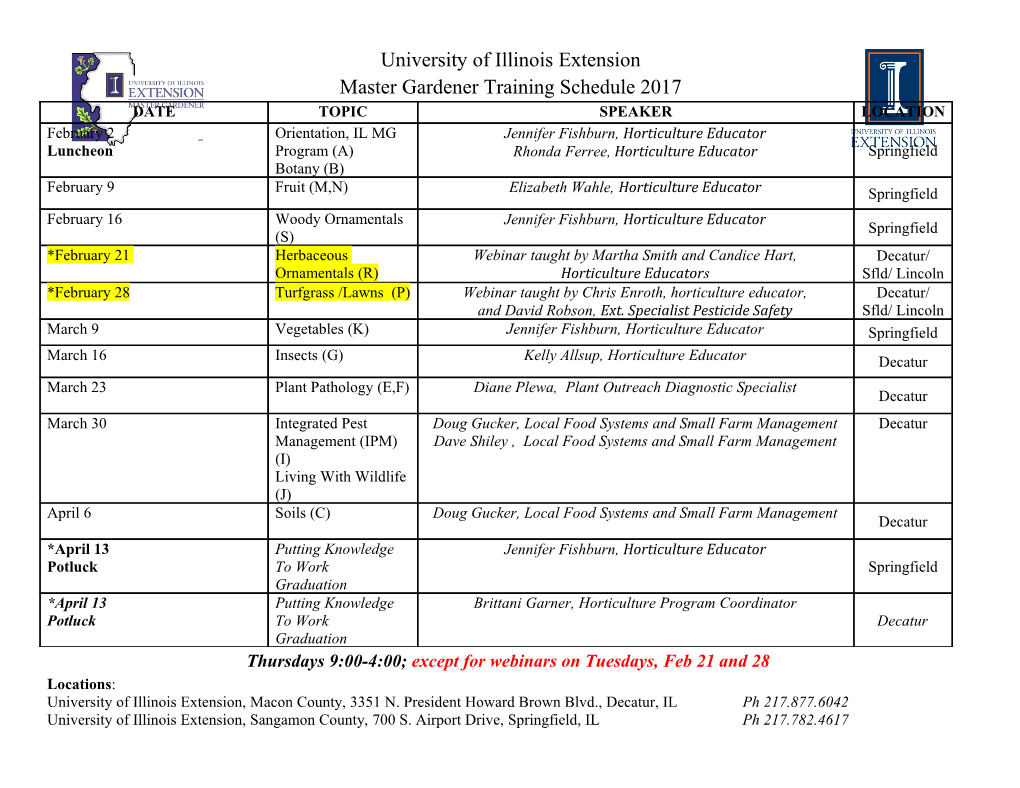
Formation of Impact Craters 17 Formation of Impact Craters The processes by which large impact craters form, and dred meters per second. In such a low-velocity impact, the the sudden releases of huge quantities of energy involved, projectile penetrates only a short distance into the target (de- cannot be duplicated in the laboratory, and, fortunately, no pending on its velocity and the nature of the target mate- such structure has formed during recorded human history. rial), and the projectile’s momentum excavates a pit that is All our knowledge about large impact structures is therefore slightly larger than the projectile itself. The projectile sur- indirect, and it has come from combining several areas of vives, more or less intact, and much of it is found in the once-separate research: theoretical and experimental stud- bottom of the pit. Such pits, sometimes called penetration ies of shock waves (for reviews and literature, see Melosh, craters or penetration funnels, are typically less than a few 1989), experimental production of small craters (e.g., Gault tens of meters in diameter. et al., 1968; Gault, 1973; Holsapple and Schmidt, 1982, 1987; Examples of these features include Brenham (Kansas), papers in Roddy et al., 1977), and geological studies of larger the many small pits made by the Sikhote-Alin (Russia) me- terrestrial impact structures (Shoemaker, 1963; Dence, 1968; teorite shower in 1947, and the pit dug by the largest piece Dence et al., 1977; Grieve and Cintala, 1981; Grieve et al., of the Kirin (China) meteorite fall in 1976. The process of 1981; Schultz and Merrill, 1981; Stöffler et al., 1988). The excavation is strictly a mechanical one, and high-pressure cratering process is complex, many details are still uncertain, shock waves are not produced. and neither calculations nor predictions can be made with In sharp contrast, a hypervelocity impact crater starts to firm confidence. But these studies provide the essential ba- form at the instant that an extraterrestrial object strikes the sis for understanding how impact craters form and for deci- ground surface at its original cosmic velocity. These impact phering the geological features they display. velocities are much greater than the speed of sound in the target rocks, and the crater is produced by intense shock waves that are generated at the point of impact and radiate 3.1. SHOCK WAVES AND outward through the target rocks. Shock waves are intense, CRATER FORMATION transient, high-pressure stress waves that are not produced by ordinary geological processes (for details, see Melosh, 1989, The general term “impact crater” is used here to desig- Chapter 3 and references therein). Peak shock pressures pro- nate a hypervelocity impact crater, the structure formed by duced at typical cosmic encounter velocities may reach sev- a cosmic projectile that is large enough and coherent enough eral hundred GPa. These pressure are far above the stress to penetrate Earth’s atmosphere with little or no decelera- levels (~1 GPa) at which terrestrial rocks undergo normal tion and to strike the ground at virtually its original cosmic elastic and plastic deformation, and the shock waves pro- velocity (>11 km/s). Such projectiles tend to be relatively duce unique and permanent deformation effects in the rocks large, perhaps >50 m in diameter for a stony object and >20 m through which they pass. for a more coherent iron one. The shock waves radiate from the impact point at high Smaller projectiles, typically a few meters or less in size, velocities that may exceed 10 km/s, much greater than the behave differently in passing through the atmosphere. They speed of sound in the target rocks. As they expand, they in- lose most or all of their original velocity and kinetic energy teract with the original ground surface to set a large volume in the atmosphere through disintegration and ablation, and of the target rock into motion, thus excavating the impact they strike the ground at speeds of no more than a few hun- crater. The formation of an impact crater by shock waves, 17 18 Traces of Catastrophe and the immediate modification of the newly formed crater well understood. One clear result is that, as one set of shock by gravity and rock mechanics, is a complex and continuous waves is transmitted outward from the interface into the tar- process. However, it is convenient to divide this process, get rocks, a complementary shock wave is reflected back into somewhat arbitrarily, into three distinct stages, each domi- the projectile (Fig. 3.1) (Melosh, 1989, Chapter 4; O’Keefe nated by different forces and mechanisms: contact and com- and Ahrens, 1993). pression, excavation, and modification (Gault et al., 1968; The shock waves transmitted into the target rocks lose see also Melosh, 1989, Chapters 4, 5, and 8). energy rapidly as they travel away from the impact point. Two factors are involved in this energy loss: (1) the expand- 3.1.1. Contact/Compression Stage ing shock front covers an increasingly larger hemispherical This stage begins at the instant that the leading edge of area with increasing radial distance, thus reducing the over- the moving projectile makes contact with the ground sur- all energy density; (2) additional energy is lost to the target face. If the target is solid rock, the projectile is stopped in a rocks through heating, deformation, and acceleration. The fraction of a second and penetrates no more than 1–2× its peak pressures of the shock waves therefore also drop rap- own diameter (Fig. 3.1) before its immense kinetic energy is idly with distance from the impact point. Theoretical mod- transferred to the target rocks by shock waves generated at els (Melosh, 1989, pp. 60–66) and geological studies of the interface between projectile and target (Kieffer and shock-metamorphosed rocks in individual structures (Dence, Simonds, 1980; O’Keefe and Ahrens, 1982, 1993; Melosh, 1989, 1968; Robertson, 1975; Grieve and Robertson, 1976; Dence et Chapter 4). al., 1977; Robertson and Grieve, 1977; Dressler et al., 1998) The general features of this conversion of kinetic energy indicate that the peak shock-wave pressure (Ps) drops expo- into shock waves have been determined from experiments nentially with the distance R from the impact point accord- α –n and theoretical studies (e.g., O’Keefe and Ahrens, 1975, 1977, ing to an equation of the form Ps R . Various field and 1993; Ahrens and O’Keefe, 1977; papers in Roddy et al., 1977; laboratory studies indicate a dependence of R–2 to R–4.5; the Melosh, 1989, Chapter 4), although many details are still not exact value of the exponent depends on projectile size and impact velocity (Ahrens and O’Keefe, 1977). On the basis of these studies, it is possible to regard the impact point as surrounded by a series of concentric, roughly hemispherical shock zones, each zone distinguished by a certain range of peak shock pressure (Fig. 3.2) and charac- terized by a unique suite of shock-metamorphic effects pro- duced in the rocks. At the impact point, peak shock-wave pressures may exceed 100 GPa (= 1000 kbar or 1 Mbar) for typical cosmic encounter velocities, producing total melting, if not vaporization, of the projectile and a large volume of surrounding target rock. Further outward, pressures of 10– 50 GPa may exist over distances of many kilometers from the impact point, producing distinctive shock-deformation effects in large volumes of unmelted target rock. At even greater distances from the impact point, the peak shock-wave pressures eventually drop to about 1–2 GPa (Kieffer and Simonds, 1980). At this point, near the eventual crater rim, the shock waves become regular elastic waves or Fig. 3.1. Contact/compression stage: shock-wave generation and projectile deformation. Theoretical cross-section showing seismic waves, and their velocity drops to that of the velocity calculated conditions immediately after the impact of a large, of sound in the target rocks (typically 5–8 km/s). These seis- originally spherical, projectile (stippled) onto a uniform target. The mic waves can be transmitted throughout the entire Earth, projectile has penetrated about half its diameter into the target, like similar waves generated by earthquakes and volcanic and intense shock waves (pressures in GPa) are radiating outward eruptions. Because of their low pressures, they do not pro- into the target from the interface. The projectile itself has become duce any permanent deformation of the rocks through which intensely compressed, and similar shock waves from the interface they pass. However, seismic waves may produce fracturing, are spreading toward the rear of the projectile. When this shock brecciation, faulting, and (near the surface) landslides, and wave reaches the rear of the projectile, it will be reflected forward the results may be difficult to distinguish from those of nor- as a tensional wave or rarefaction, unloading the projectile and mal geological processes. allowing it to transform, virtually instantaneously, into melt and The duration of the contact/compression stage is deter- vapor. The original model, developed for large lunar impact events (O’Keefe and Ahrens, 1975), represents conditions about 1 s after mined by the behavior of the shock wave that was reflected the impact of a 46-km-diameter anorthosite projectile at 15 km/s back into the projectile from the projectile/target interface onto a gabbroic anorthosite target, but similar conditions will be (Fig. 3.1) (Melosh, 1989, pp. 57–59). When this shock wave produced by smaller impacts and other material compositions. reaches the back end of the projectile, it is reflected forward (Modified from Melosh, 1989, Fig. 4.1a, p. 47.) into the projectile as a rarefaction or tensional wave (also Formation of Impact Craters 19 Fig.
Details
-
File Typepdf
-
Upload Time-
-
Content LanguagesEnglish
-
Upload UserAnonymous/Not logged-in
-
File Pages14 Page
-
File Size-